Hormones are unique molecules produced by specific cells or tissues known as endocrine glands. These molecules are released from their cells of origin and are carried to their respective target cells by a fluid medium, typically blood.
Introduction to Hormone Action
The hormones act as biochemical signals, stimulating a specific reaction in the target cells, which typically serves the overall health and well-being of the organism. This signaling process is integral to the body’s homeostatic response, which is the way the body adjusts to changes in its internal and external environments. In essence, hormones function as messenger molecules, transmitting vital information from one part of the body to another.
Once a hormone reaches its target cell, it triggers a sequence of events within the cell, often activating or inhibiting specific molecules. This leads to the generation of ‘second’ messengers within the cell. However, it’s important to differentiate hormones from other types of molecules like nutrients or waste products. For example, the liver produces glucose, a critical energy substrate. But, since glucose is a nutrient, it doesn’t fit the strict definition of a hormone, despite being a product of internal secretion.
Expanding Our View of Hormone Action
Conversely, the liver also produces other types of molecules that are neither simple nutrients nor waste products. These ‘first’ messenger molecules are indeed hormones, as they are released into the bloodstream and carried to various target tissues throughout the body. In this respect, the liver functions as an endocrine tissue. Hepatocytes are part of a larger group of tissues referred to as non-traditional endocrine tissues.
Additionally, the definition of hormones excludes molecules that are secreted from nerve endings and travel across small gaps or synapses between neurons. These molecules are known as neurotransmitters or neuromodulators. That being said, certain neurons, particularly those within the central nervous system (CNS), can also act as endocrine cells, producing hormones. The study of these neurons and their secretions is a rapidly growing field known as neuroendocrinology.
The characterization of a molecule as a hormone can sometimes be challenging. For instance, cytokines are proteins produced by cells of the immune system. They carry out communication functions between different cells and are transported by the blood to have broad effects in the body, including within the CNS. Thus, they act in a similar way to hormones. Cytokines include interleukins and other ‘factors’ such as tumor necrosis factors and interferons, all of which are components of the immune system.
Answering the Question, What is a hormone?
One approach to determining if a molecule is a hormone involves examining whether it has specific receptors on its target cells. This method effectively eliminates simple nutrients and waste products, which generally lack such receptors. In some cases, the discovery of a new protein through gene product investigation may hint at a receptor protein. Until a binding molecule or ligand (a substance that binds to a receptor, like a hormone) is identified for this receptor, it’s often referred to as an “orphan receptor.”
By definition, hormones are biochemical substances produced by certain cells, which are then released into the bloodstream to influence distant target cells. It’s plausible that hormones can also be released into, or enter, other circulating fluids such as cerebrospinal fluid, seminal fluid, amniotic fluid, and lymph. These fluids are essentially composed of water with various solutes and ions, and perhaps cells and cell fragments.
Hormones, as chemicals, may exhibit different properties based on their composition. Some hormones, like amino acids, peptides, and proteins, are hydrophilic (water-loving) and can be considered lipophobic (lipid-averse). On the other hand, hormones like steroids are lipophilic (lipid-loving) or hydrophobic (water-averse).
Mechanism of Action of Hormones
The chemical characteristics of a hormone significantly influence its synthesis, storage, release from the endocrine cell, transport in the fluid medium, and its mechanism of action. Interestingly, some molecules generally considered gaseous have been shown to play an endocrine role. However, because they are short-lived in the general circulation, they likely only impact cells near their production sites. Nitric oxide serves as a prime example of such a molecule. It’s found in many different tissues and generally affects local cells.
While most hormones influence distant target cells in their endocrine roles, others like nitric oxide can affect nearby cells, exhibiting what is known as a paracrine effect. Some hormones may even influence their own cells of production, regulating their synthesis, storage, and release processes. This phenomenon is termed an autocrine effect.
Another term used to describe the location of action for some hormones is “cryptocrine”. This term pertains to molecules, such as hormones, that a cell produces and which act within a confined space linked to its production cell. An example of this cryptocrine activity is the Sertoli cell in the testis, which produces factors that affect developing spermatids within the cell’s enclosed environment.
Adjacent cells often establish unique physical connections that enable them to “communicate”. One such example includes gap junctions, where the membranes of two cells make direct contact. These junctions contain membrane proteins, known as connexins, which form channels or connexons. These connexons permit the transfer of small molecules like ions and nutrients between the two cells.
Another example involves the creation of dynamic tight junctions, which temporarily bind adjacent cells together. These tight junctions, composed of transmembrane proteins, can temporarily trap extracellular fluid between the cells. Any molecule secreted by one cell into this minuscule extracellular space can reach a high local concentration. If receptors for that molecule exist on the neighboring cell, an effect can be induced that wouldn’t be possible if the hormone had to travel through the general circulation to reach the second cell. This longer route would result in considerable dilution of the hormone concentration.
The primary hormones, along with their chemical characteristics and main sites of production, are well documented. It’s important to note that many hormones and some endocrine glands have alternate names that can be used interchangeably. The differing terminologies will be discussed in more detail in the relevant sections that delve into individual glands and their corresponding hormones.
Hormones vs. neurotransmitters
Hormones and neurotransmitters are vital in maintaining the body’s homeostasis in response to various internal and external stimuli. Both of these systems use chemical ‘messengers’ and share certain resemblances, but there are also significant differences between them.
When stimulated, neurons generate neurosecretions that are usually released at the nerve terminals, predominantly through synaptic gaps between neurons, acting as neurotransmitters. However, some neurons release these neurosecretions into the bloodstream, acting as hormones. Recent research suggests that some of these neurosecretory molecules could be transported to more distant target cells via cerebrospinal fluid or general brain extracellular fluid, which also justifying their classification as hormones.
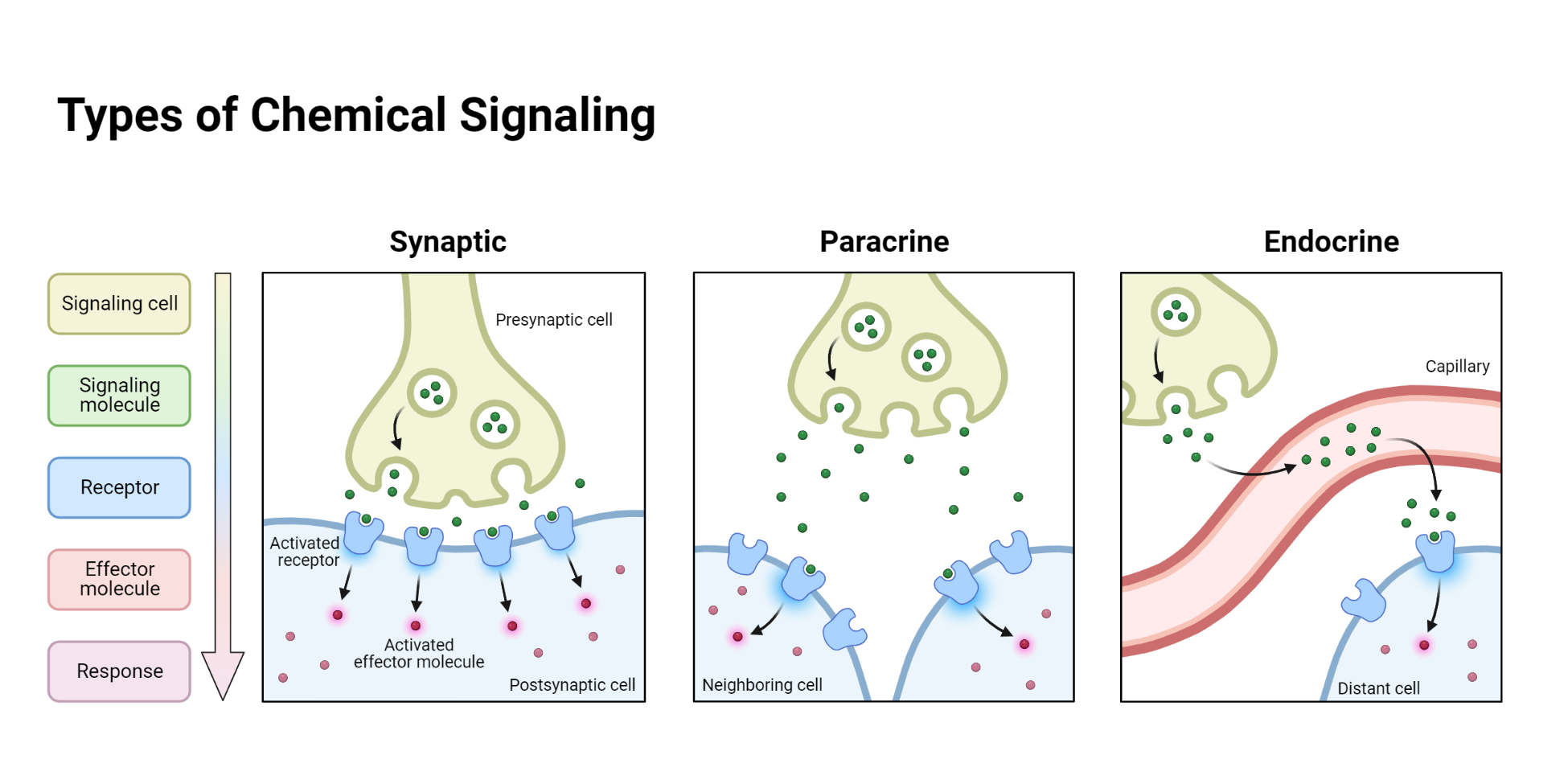
A key component of the brain that is crucial in controlling our internal environment is the hypothalamus. The hypothalamus influences numerous peripheral endocrine glands. Some of its neurons release their neurosecretions into a specialized bloodstream, which transports them to a ‘mediating’ endocrine gland, the pituitary gland. Therefore, the hypothalamus can be accurately seen as both part of the central nervous system (CNS) and the endocrine gland itself.
While neural and endocrine systems are integral to the body’s diverse activities, the differences between them also warrant discussion. Here are some of the shared characteristics and distinguishing factors:
Similarities:
- Both neurotransmitters and hormones involve the release of specific chemicals.
- Neurotransmitters are generally small molecules such as acetylcholine, amino acids, or amino acid-derived molecules, while hormones can also be amino acid-derived, but also include polypeptides, steroids, and larger proteins and glycoproteins. However, this distinction is becoming less clear-cut as we now know that neurons can also produce polypeptides and steroids.
- Both neurotransmitters and many hormones are discharged from vesicles into the surrounding fluid by similar mechanisms involving calcium ions and an expulsion system called exocytosis.
- Their mechanisms of action are generally similar, often involving either ion channels or G protein-related receptors.
Key Differences:
Differences between these two regulatory systems are also quite distinct. One of the main differences is in the delivery of their message: neurotransmitters act in a rapid and localized manner, while hormones tend to have slower, more generalized effects. Another crucial distinction is the fact that neurotransmitters are primarily involved in neurotransmission across synaptic gaps, while hormones are secreted into the bloodstream and affect distant target cells. Furthermore, neurotransmitters are metabolized and removed rapidly, whereas hormones can persist in the circulation for a longer period.
General Classification of Hormones
Hormone subtype | Examples (Endocrine Gland) |
Amino acid or amino acid derived | Thyroxine and tri-iodothyronine (thyroid gland), Adrenaline and noradrenaline (adrenal medulla), Dopamine (hypothalamus) |
Polypeptide | Insulin (Pancreas) Glucagon (Pancreas) Vasopressin (Neurohypophysis) Oxytocin (Neurohypophysis) Corticotrophin (Adenohypophysis) Calcitonin (Thyroid parafollicular cells) Parathormone (Parathyroid glands) Somatostatin (Hypothalamus) Corticotrophin-releasing Hormone (Hypothalamus) Thyrotrophin-releasing hormone (Hypothalamus) Gonadotrophin-releasing Hormone (Hypothalamus) Inhibin (Testis, ovary) Activin (Ovary) Atrial natriuretic peptide (Heart) |
Protein | Somatrotrophin (Adenohypophysis) Prolactin (Adenohypophysis) Erythropoietin (Kidney) Cytokines (Immune cells) Leptin (Adipose tissue) Ghrelin (Stomach) |
Glycoproteins | Thyroxine and tri-iodothyronine (thyroid gland) Adrenaline and noradrenaline (adrenal medulla), Dopamine (hypothalamus) |
Steroids | Aldosterone (Adrenal) Cortisol (Adrenal) Testosterone (Testis) 17β-estradiol (Ovary) Estrone (Ovary) Progesterone (Ovary) Calcitriol (Kidney) |
Next, we will explore each major subtype of endocrine hormones. Lets start with polypeptide and protein hormones.
Proteins and Polypeptide Hormones
Polypeptide and protein hormones consist of chains of amino acids. Polypeptides typically comprise between 2 to 100 amino acids, while proteins are made of longer chains, often more than 100 amino acids in length. Some proteins are glycosylated, which means they have carbohydrate residues attached to them, and these are known as glycoproteins. These hormones can also be composed of more than one chain linked together.
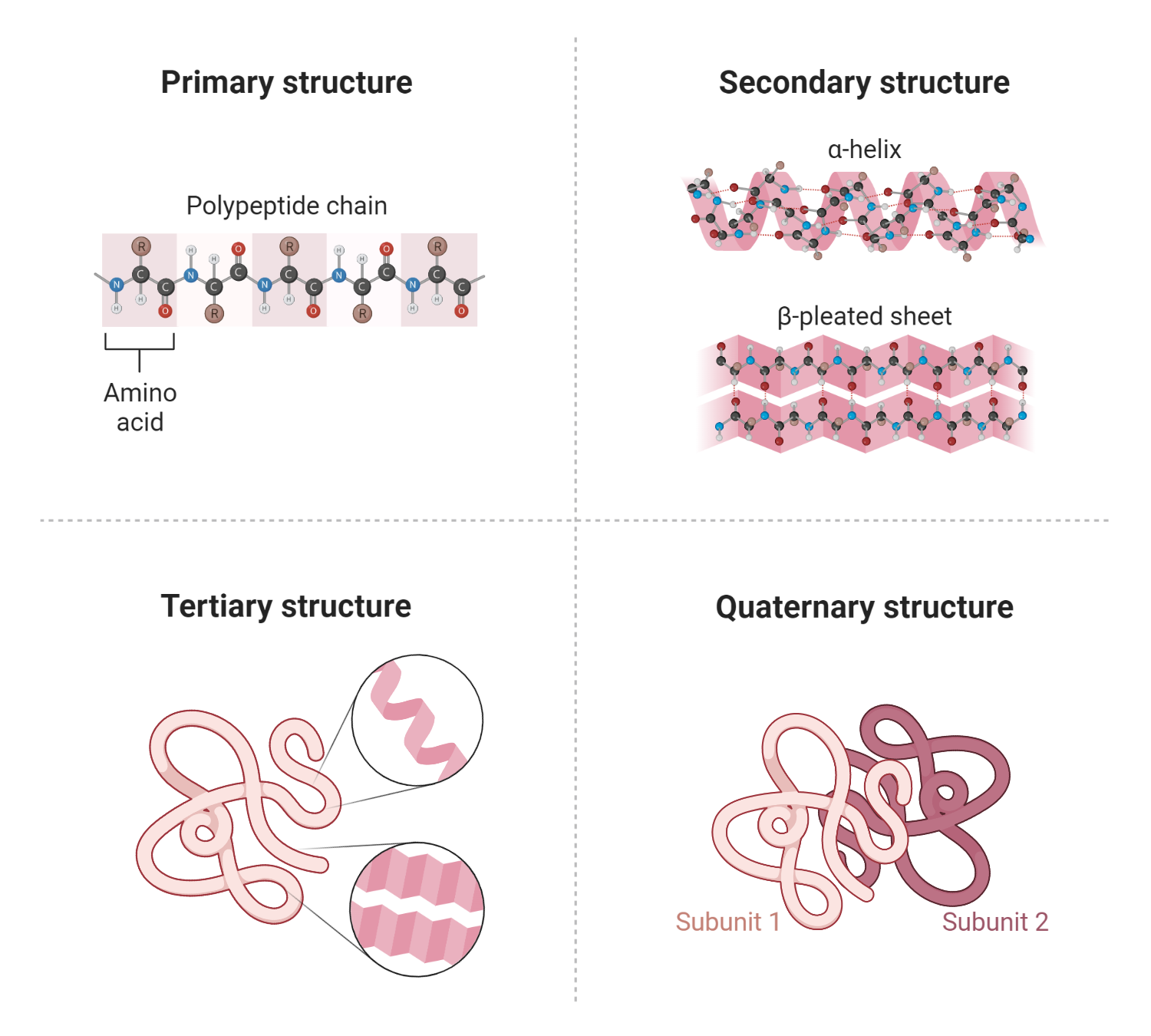
The process of these hormones’ synthesis begins as larger precursor molecules called prohormones, which are subsequently cleaved to form different products, including the intended hormones. The endocrine cells responsible for the production and exportation of these molecules contain several advanced intracellular organelles, including the endoplasmic reticulum, the Golgi complex, and secretory granules.
The synthesis process initiates with the activation of a specific gene on a chromosome within the nucleus of the endocrine cell by a transcription-stimulating factor. When this gene is activated, a new protein molecule is synthesized, a process called gene expression.
The gene, made up of deoxyribonucleic acid (DNA), carries the code for an amino acid chain. Transcription of the gene code sequence into a corresponding molecule of messenger ribonucleic acid (mRNA) is facilitated by an enzyme known as RNA polymerase.
Not all parts of a gene are transcribed into protein. Each gene is composed of exons, which do transcribe into proteins, and introns, which do not. As the complementary mRNA strand is synthesized within the nucleus, the introns are removed by enzymes known as small nuclear ribonucleoproteins (snRNPs). This process results in a functional strand of mRNA which passes through a pore in the double membrane comprising the nuclear envelope.
To transform the mRNA molecule into a new protein, the coded information in the nucleotide sequence needs to be translated into a complementary sequence of amino acids. This translation process takes place in ribosomes, which are either freely present in the cytoplasm or bound to the membrane of the rough endoplasmic reticulum (RER).
The synthesis of a polypeptide hormone generally starts with the formation of an initial larger protein called a pre-prohormone. This molecule moves from its ribosome through the membrane of the RER. This transfer into the RER follows recognition of the initial segment of the molecule, which is known as the signal peptide. This highly intricate process underscores the complexity and precision of the body’s biochemical machinery.
Upon entering the sacs or tubules of the rough endoplasmic reticulum (RER), a segment known as the signal peptide is cleaved off the initial larger protein (pre-prohormone), resulting in the formation of a prohormone precursor. This precursor then proceeds from the RER into the neighboring Golgi complex where it may undergo further modifications.
For instance, in the Golgi complex, a prohormone could be enzymatically cleaved to generate more than one protein or polypeptide, or it might be glycosylated, which means attaching carbohydrate residues to it, or subjected to other modifications. The concluding stage in peptide hormone synthesis involves packing the breakdown products of the prohormone into vesicles that originate from the Golgi membrane.
In some cases, a single prohormone precursor can yield various peptide breakdown products, which include the known biologically active hormone. Although many of these peptides do not have identified functions yet, ongoing research is likely to reveal their roles or biological activities within the body. These vesicles, ubiquitously present in protein hormone-secreting endocrine cells, act as important storage units for hormones.
Interestingly, every eukaryotic cell within an individual contains identical genetic material, with particular genes normally being expressed in specific tissues. This implies that non-endocrine tissues could possibly produce abnormal polypeptide hormones should the relevant gene expression be activated.
Such aberrations can occur when a cell becomes abnormally stimulated by a specific chemical (carcinogen), leading to the inappropriate activation of the gene. Consequently, some non-endocrine tissue tumors, such as those in the lung, can turn into abnormal or ectopic sources of protein hormones. Beyond the direct effects of tumor growth itself, complications can also stem from the unregulated, and often exceedingly high, circulating levels of the bioactive hormone being produced.
Steroid Hormones
Steroid hormones constitute the other significant group of hormones originating from a common initial precursor: cholesterol. Because of their lipophilic or fat-loving nature, these steroids can readily diffuse out of the endocrine cells where they are synthesized.
For a long time, it was assumed that these hormones exited the cell by simply diffusing through the lipid cell membrane. However, recent research indicates the existence of specific transporters within the cell membrane, which would undeniably facilitate this process. As one might expect, the minimal steroid hormone is retained within its synthesizing cell because it typically diffuses out immediately. Therefore, it is generally synthesized on demand, that is, when the endocrine cell receives the appropriate stimulation.
The synthesis process of steroid hormones involves the activation of particular intracellular enzymes which catalyze chemical conversions, such as hydroxylation and aromatization, transforming precursors into the final bioactive hormone molecules.
Similar to endocrine cells that produce peptide and protein hormones, the cells that synthesize steroid hormones continually receive stimulatory and inhibitory signals concerning the internal environment from various sources. These signals are integrated in some manner to generate the ultimate endocrine response. In these cells, the various enzymes that convert earlier molecular stages into the final bioactive molecule are the primary targets for these signaling pathways.
Amino Acid Based Hormones
Certain crucial hormones, such as the iodothyronines of the thyroid gland and the catecholamines of the adrenal medulla, are derived from amino acids. These hormones have distinct synthesis pathways, which will be discussed in more detail in corresponding sections of this text. An amino acid serves as the initial precursor molecule and undergoes enzymatic modifications to form the final bioactive hormone molecule.
The general properties of these amino acid-derived hormones vary and are unique to each hormone type. Typically, they are stored within the endocrine cells or in specialized structures known as follicles. When transported in the bloodstream, they can travel freely or remain protein-bound. The receptors for these hormones are located on the plasma membranes of their target cells, where the hormones bind to exert their effects.
Hormone Storage Process
The molecular makeup of a hormone greatly influences whether it gets stored before being released. This aspect of storage also tends to differ between the two primary hormone categories. Particularly, protein and polypeptide hormones present a distinctive feature in terms of storage.
Concerning protein and polypeptide hormones, they are typically stored within cells in compartments known as vesicles. This occurs during their synthesis process. Therefore, when a cell that produces these hormones is triggered, two things happen. First, the synthesis pathway that generates these hormones at the genetic level is activated. Second, the vesicles that hold the stored hormones are prompted to move to the cell’s outer membrane.
This characteristic is crucial to understanding that the endocrine cell, when stimulated, can perform dual functions. It can generate new protein and polypeptide molecules for future needs, and it can also expel hormones that were synthesized earlier and stored in the vesicles. The activation of separate intracellular pathways (also known as second messenger pathways) for these tasks can occur concurrently or independently, based on the nature of the triggering stimulus.
Another point worth mentioning is that an additional reserve of preformed protein and polypeptide hormones can exist within the bloodstream. Certain hormones, after their release, attach to plasma proteins and circulate in a balanced state between their bound and free (unattached) forms. Growth hormone, for instance, binds with numerous plasma proteins in the bloodstream. This isn’t a standard mechanism for protein and polypeptide hormones, though. Generally, these hormones are stored in vesicles inside cells and get released when the endocrine cell receives the appropriate signal. Other potential sources for these hormones can be elements within the blood, such as platelets. For instance, the hormone vasopressin is found in platelets in considerable amounts and can be discharged when these platelets are disturbed. This can be a beneficial response in specific situations, like at a hemorrhage site, since vasopressin triggers vasoconstriction.
Hormones derived from amino acids, such as iodothyronines and catecholamines, are stored within endocrine glands. Like their larger counterparts, catecholamines such as epinephrine (adrenaline) and norepinephrine (noradrenaline) are stored in vesicles and released through exocytosis. Iodothyronines are stored in an unconventional extracellular form within follicles, synthesized by thyroid follicular cells. When these cells receive the appropriate stimulation, the iodothyronines are reabsorbed into the cells and eventually released into the bloodstream. Catecholamines circulate freely, whereas iodothyronines mainly circulate bound to plasma proteins.
Steroid hormones, due to their lipophilic nature, are not typically stored in bioactive form within endocrine cells. They are mostly synthesized when needed. However, all cells contain some cholesterol, the precursor molecule for steroid hormones, hinting at a tiny amount of potential storage.
This doesn’t mean that such hormones are absent from circulation until endocrine cells are stimulated to synthesize and release them. Steroid hormones are primarily transported in the blood attached to plasma proteins. A dynamic balance exists between free (biologically active) hormones and those attached to plasma proteins. This balance ensures that most hormones at any given time are ‘stored’ in the blood as the inactive, bound component.
The equilibrium can be described in terms of concentrations of the components:
[free hormone H] + [plasma protein P] ↔ [protein-bound hormone HP]
At equilibrium, the reaction can be expressed as Ka = [HP]/[H][P] where Ka is the association constant.
This balance not only provides a consistent source of hormone in circulation in ‘storage form’ as the protein-bound component but also means that if the equilibrium is disturbed, the components automatically rearrange to restore the balance. For example, in areas of the body where there may be an increased presence of hormone receptors, the bioactive free component would bind to its receptors, causing a local disequilibrium. The physical forces at work would result in a transfer of hormones from the protein-bound to the free state to restore the balance.
Plasma proteins involved in hormone transport fall into two main categories: those specific for certain hormones and those that aren’t specific but are present in high amounts and can absorb large quantities of hormones. The defining trait of a plasma protein is its affinity for binding hormones and its capacity for binding. High-affinity plasma proteins, such as those for glucocorticoids (e.g., cortisol), can transport large amounts of these hormones. They are usually globulins (e.g., cortisol-binding globulin).
Albumins, on the other hand, form the largest plasma protein component of the blood. Even though they don’t have a high affinity for any particular hormone, due to their abundance, they have a relatively high capacity for hormone transport and can carry a significant amount
It’s important to remember that both cortisol and aldosterone, steroid hormones produced by the adrenal cortex, also bind to cortisol-binding globulin, hinting at some overlap in hormone binding.
Another crucial aspect to consider, particularly in a clinical setting, is that plasma proteins are produced by the liver, and their synthesis can be affected by certain hormones like estrogens. Therefore, conditions that impact liver function or the production of estrogens can influence the blood’s capacity to bind proteins.
Release of hormones
Hormone release is another area where the production of hormones can be regulated. Depending on the category, hormones are either released from a pre-existing store or generated when needed.
Protein and polypeptide hormones are generally stored within the cells that produce them in structures called vesicles. Their release requires these vesicles to move to the cell’s outer plasma membrane and merge with it, which allows the vesicle’s contents to be expelled. This process is known as exocytosis. The cell’s cytoskeleton, a dynamic, lattice-like structure of protein filaments, is integral to this process as it aids in moving the vesicles to the outer plasma membrane.
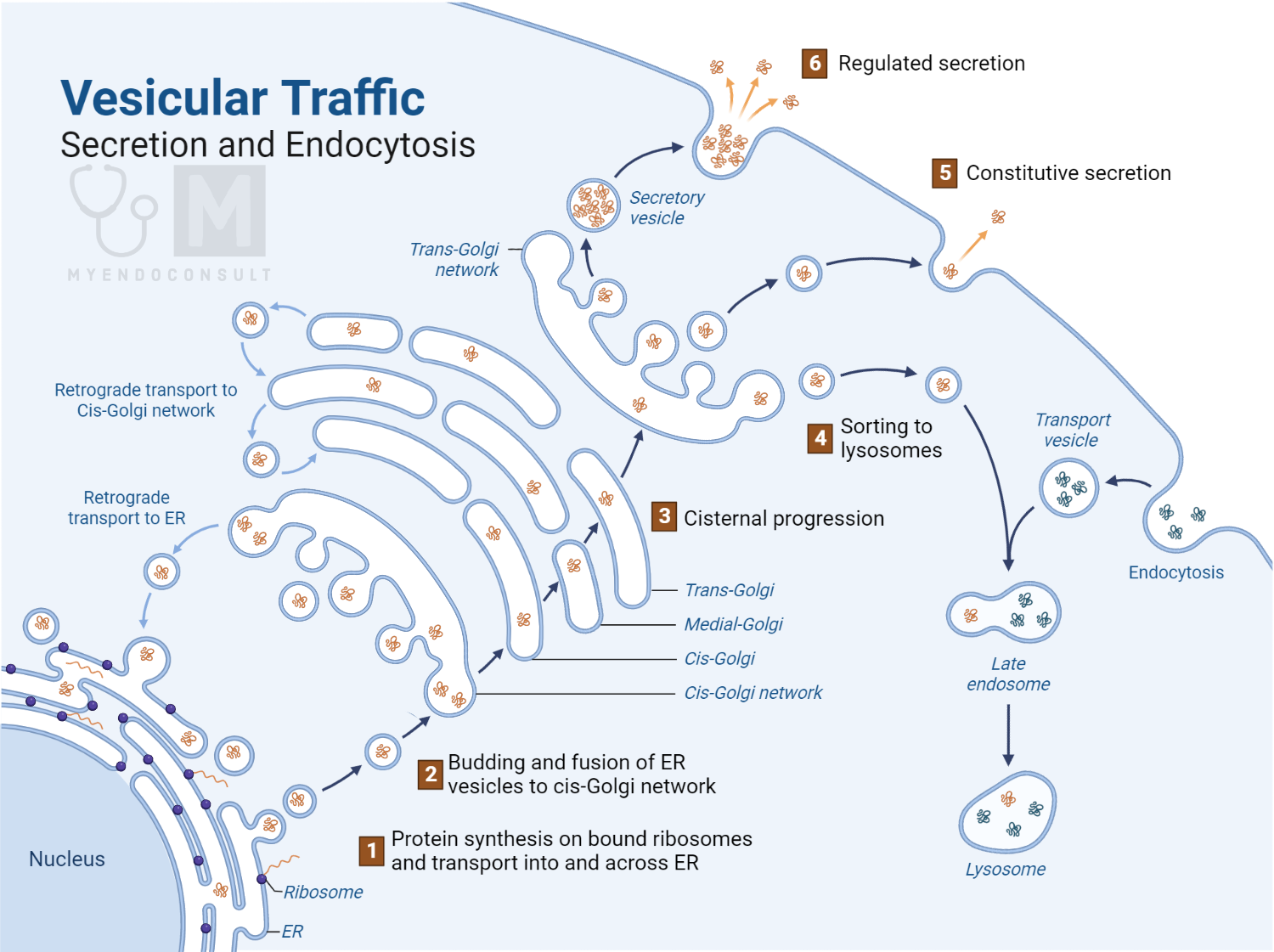
Vesicle ‘trafficking’, or the movement of vesicles from the cell’s interior to the outer plasma membrane, is considered to involve the movement of vesicles along these filaments towards the plasma membrane. Exocytosis only happens when the filaments beneath the membrane break down, enabling the vesicles to reach the membrane. Various other molecules play a crucial role in this process, like recognition and anchoring proteins. While our understanding of this process is still developing, the culmination of exocytosis is the fusion of the vesicle membrane with the plasma membrane, leading to the expulsion of the vesicle’s contents.
The amino acid-derived hormones, such as catecholamines, are stored in vesicles within the adrenal medulla cells, making their secretory process similar to protein and polypeptide hormones. In contrast, steroids and other lipophilic hormones, due to their nature, are not stored in vesicles but are synthesized on demand. Their release into the bloodstream is thought to result from passive diffusion through the cell membrane, but there may be other mechanisms involved, though this is speculative at present.
Once released into the circulation, a hormone can be transported freely, bound to a plasma protein, or transported within a cell or cell derivative in the blood. It’s generally believed that the free, unbound hormone represents the bioactive fraction. Some hormones, like growth hormone, have binding proteins, and a significant portion of some hormones, like vasopressin, is transported within the platelets.
When a hormone reaches its target cell, it needs to recognize it and influence it in some way, a process called signal transduction. This process begins with the recognition of the target cell by the hormone, which requires a specific molecule (receptor) on the target cell to which the hormone can bind. The receptor can be located in the plasma membrane of the target cell.
Receptors, which are usually located within the cell, in the cytoplasm or nucleus, or in the cell’s plasma membrane, are essential for a hormone to identify and interact with its target cell. Although a receptor is ideally specific to a particular hormone molecule, it’s more common for it to bind with other chemically similar molecules or ligands. Hundreds of these receptor proteins have been identified so far.
There are several important aspects regarding hormones and their receptors:
- A single hormone might bind with different receptors, with each receptor type distributed among various tissues, thereby allowing the hormone to affect different target cells and tissues.
- Similarly, a receptor might not be specific to a single hormone and might bind with other ligands with varying degrees of affinity.
- Different receptor subtypes are likely linked to varied intracellular second messenger pathways, which can induce diverse responses in different target cells.
- The effect of a hormone on a target tissue can be influenced by both the concentration of the bioactive hormone near the target cell and the number of receptors present. So, a disorder resulting from the loss of a hormone’s activity could be due to a lack of hormone production or a deficiency or abnormality in its receptors.
To prevent a specific stimulated intracellular pathway from remaining indefinitely active, there needs to be cellular regulatory mechanisms to disconnect the hormone-receptor interaction and deactivate the hormone.
Protein and polypeptide hormones, being averse to lipids, are unlikely to penetrate the target cell membranes without specific transport systems. Hence, these hormones generally have their receptors embedded in the plasma membranes of their target cells. A typical receptor of this sort would be a transmembrane protein with an extracellular domain for the hormone molecule to attach to, a membrane-spanning segment, and an intracellular domain associated with a specific transduction process.
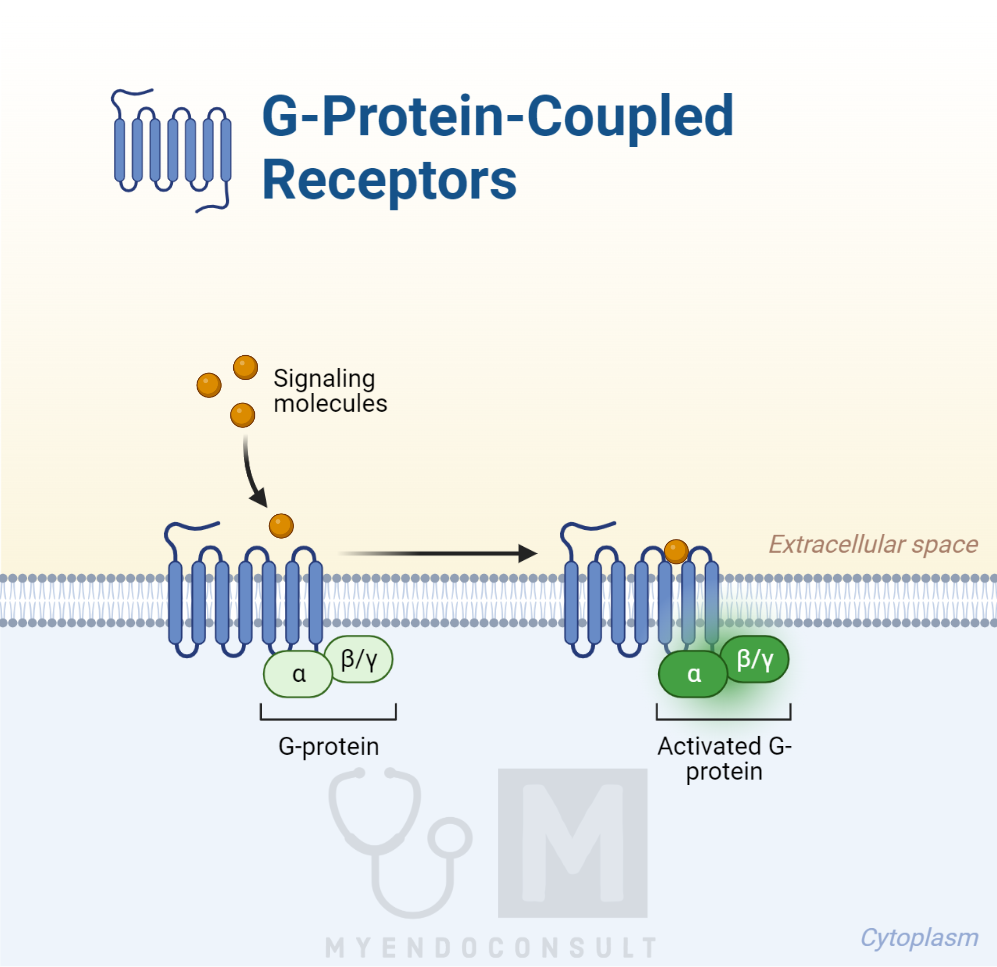
One widespread group of such receptors is the seven-transmembrane receptor group, named for their seven membrane-spanning helices. These receptors connect to a specific intracellular protein known as a G protein, which triggers the intracellular transducing mechanism, activating the cell. These receptors are hence called G-protein–coupled receptors (GPCRs). They have intricate regulatory mechanisms, and over 30 intracellular molecules, known as regulators of G protein signaling (RGSs), and other molecules, called activators of G protein signaling (AGS), can influence them.
There are also receptors with a single strand spanning the membrane. These receptors have an extracellular domain with hormone-binding capability and an intracellular domain with inherent enzyme activity when activated.
This type of receptor can exist as homodimers (double single-spanning receptors) or as heterodimers, where two different receptors link together. When the hormone or other ligand binds to the extracellular domain, the receptor’s conformation changes, activating the associated intracellular enzyme or G protein and transducing the hormone’s ‘message’ to the cell’s interior.
Reference(s)
Stárka L, Dušková M. What is a hormone? Physiol Res. 2020 Sep 30;69(Suppl 2):S183-S185. doi: 10.33549/physiolres.934509. PMID: 33094616; PMCID: PMC8603735.
Kindly Let Us Know If This Was helpful? Thank You!