What is Gluconeogenesis
Gluconeogenesis is a critical metabolic pathway that allows the body to produce glucose from non-carbohydrate sources. This process occurs primarily in the liver and, to a lesser extent, in the kidneys and small intestine. While carbohydrates are the body’s preferred source of energy, during periods of low carbohydrate availability, such as fasting, starvation, or intense exercise, the body must find alternative sources of glucose.
The term “gluconeogenesis” literally means the creation (genesis) of new (neo) glucose. It’s essentially the reverse process of glycolysis, a metabolic pathway that breaks down glucose to produce energy. However, gluconeogenesis isn’t a simple reversal of glycolysis, but involves a series of eleven enzymatically catalyzed reactions, three of which are unique to the pathway, enabling the production of glucose from compounds such as lactate, pyruvate, glycerol, and amino acids.
Brief Overview of its Significance
The significance of gluconeogenesis is rooted in its role as a vital adaptive response to maintain blood glucose levels, the primary source of energy for the brain and red blood cells.
During prolonged periods of fasting or intense exercise when glucose intake is not sufficient to meet the body’s needs, gluconeogenesis kicks in to maintain blood glucose homeostasis. This is especially crucial for the brain, which primarily relies on glucose for energy and proper functioning. Gluconeogenesis also serves to replenish the body’s stores of glycogen, the storage form of glucose, in the liver and muscles.
Gluconeogenesis isn’t only crucial for individuals during periods of fasting or intense physical exertion. It also plays a fundamental role in various health conditions. For instance, in individuals with type 1 diabetes, dysregulation of gluconeogenesis can lead to hyperglycemia. On the other hand, in malnourished individuals or those with anorexia nervosa, increased gluconeogenesis can lead to excessive weight loss and related health problems.
Thus, understanding gluconeogenesis is not just important in the context of metabolic biology, but it also has broader implications for health, disease, and, potentially, therapeutic interventions.
The Biochemical Pathway of Gluconeogenesis
Gluconeogenesis is a vital metabolic pathway primarily occurring in the liver and, to a lesser extent, the kidneys. This process transpires mainly in the cytoplasm of the cell, with some steps taking place in the mitochondria. Let’s walk through the critical steps of gluconeogenesis, noting the participating enzymes and key intermediates.
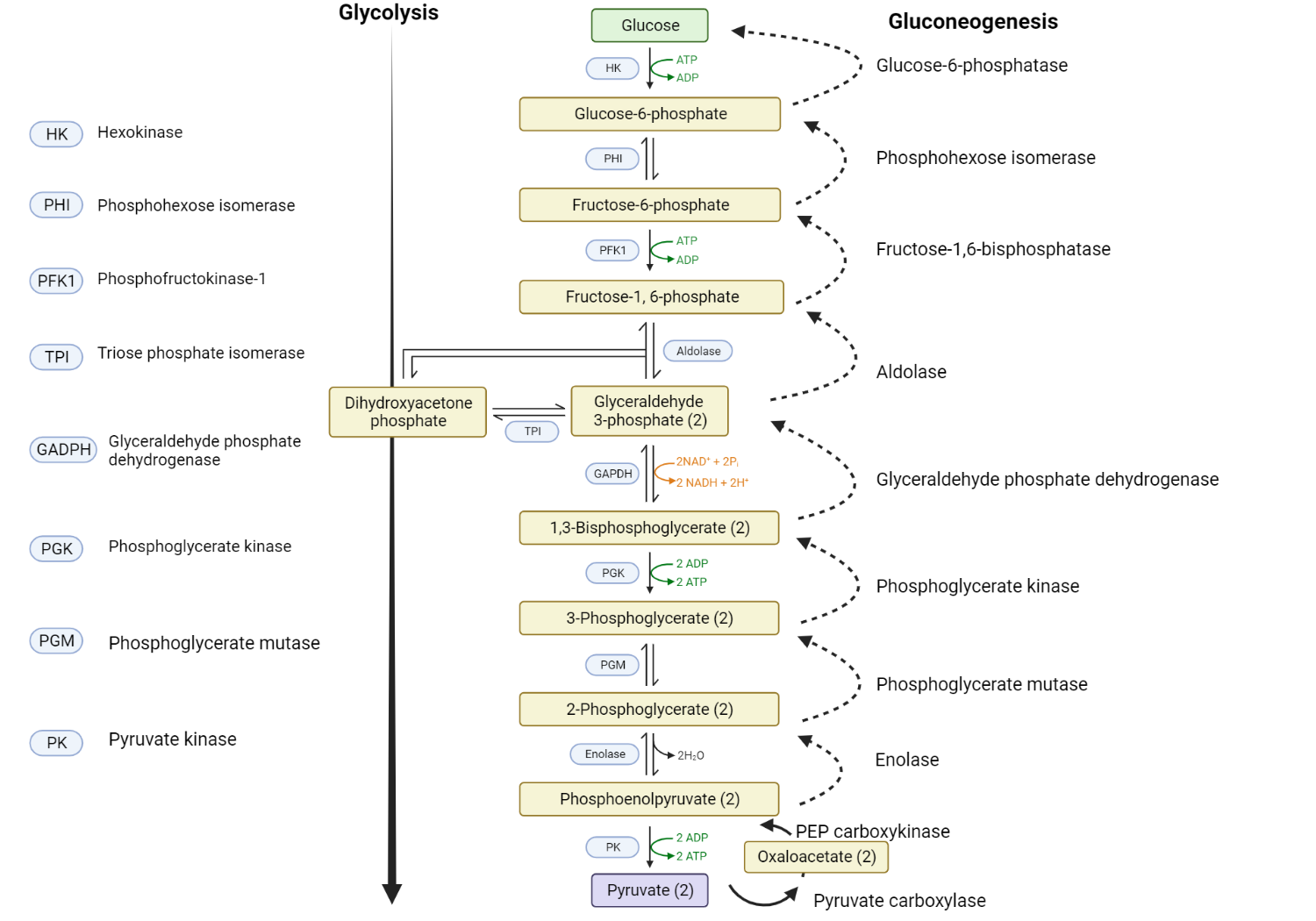
1. Pyruvate to Oxaloacetate: This is the first step in gluconeogenesis and occurs within the mitochondria. Here, the three-carbon molecule pyruvate is carboxylated (a carbon dioxide is added) by the enzyme pyruvate carboxylase, resulting in a four-carbon molecule known as oxaloacetate. This reaction requires energy, which is provided by ATP.
2. Oxaloacetate to Phosphoenolpyruvate: This step happens in two stages and is mediated by the enzyme phosphoenolpyruvate carboxykinase (PEPCK). The first stage happens in the mitochondria, where oxaloacetate is decarboxylated (a carbon dioxide molecule is removed) and converted into an unstable molecule that is quickly converted into phosphoenolpyruvate (PEP). This reaction also requires energy, provided by GTP. After formation, PEP is transported to the cytoplasm where the rest of gluconeogenesis occurs.
3. Conversion of PEP to Fructose-1,6-bisphosphate: The steps from PEP to fructose-1,6-bisphosphate essentially follow the reverse of glycolysis, involving a series of reactions that are catalyzed by the same enzymes used in glycolysis.
4. Fructose-1,6-bisphosphate to Fructose-6-phosphate: This is another critical regulatory step of gluconeogenesis, which takes place in the cytoplasm. The enzyme fructose-1,6-bisphosphatase-1 catalyzes the hydrolysis of fructose-1,6-bisphosphate to fructose-6-phosphate, bypassing a step in glycolysis.
5. Fructose-6-phosphate to Glucose-6-phosphate: This conversion occurs via the enzyme phosphoglucoisomerase, following the reverse of the glycolytic pathway.
6. Glucose-6-phosphate to Glucose: The last step of gluconeogenesis happens primarily in the liver and kidneys. The enzyme glucose-6-phosphatase catalyzes the hydrolysis of glucose-6-phosphate to glucose, which can then be released into the bloodstream to maintain blood glucose levels.
In summary, the key enzymes of gluconeogenesis that bypass the irreversible steps of glycolysis are pyruvate carboxylase, PEPCK, fructose-1,6-bisphosphatase-1, and glucose-6-phosphatase. The key intermediates in the process include pyruvate, oxaloacetate, PEP, 3-phosphoglycerate, 1,3-bisphosphoglycerate, glyceraldehyde-3-phosphate, fructose-1,6-bisphosphate, fructose-6-phosphate, and glucose-6-phosphate.
Energy Balance and Regulation of Gluconeogenesis
The process of gluconeogenesis is an energy-demanding one, requiring ATP and NADH for specific steps of the pathway. Regulation of this pathway is crucial, as it must be finely tuned to the body’s energy needs, and this is accomplished through hormonal control, primarily by insulin, glucagon, and cortisol.
Role of ATP and NADH
ATP (adenosine triphosphate) provides the necessary energy for several steps in the gluconeogenesis pathway. The first step, the conversion of pyruvate to oxaloacetate by pyruvate carboxylase, is driven by ATP. Similarly, the conversion of oxaloacetate to phosphoenolpyruvate by phosphoenolpyruvate carboxykinase is powered by GTP, which is another high-energy molecule similar to ATP.
NADH (Nicotinamide adenine dinucleotide + Hydrogen) is involved in the conversion of 1,3-bisphosphoglycerate to glyceraldehyde-3-phosphate. This step is essentially the reverse of a step in glycolysis and requires the reducing power provided by NADH.
Role of Insulin, Glucagon, and Cortisol
The hormones insulin, glucagon, and cortisol play key roles in the regulation of gluconeogenesis:
Insulin: Insulin is released in response to high blood glucose levels, such as after a meal. It promotes the uptake and storage of glucose in cells and inhibits gluconeogenesis. Insulin downregulates the enzymes involved in gluconeogenesis, thus reducing the production of glucose from non-carbohydrate sources.
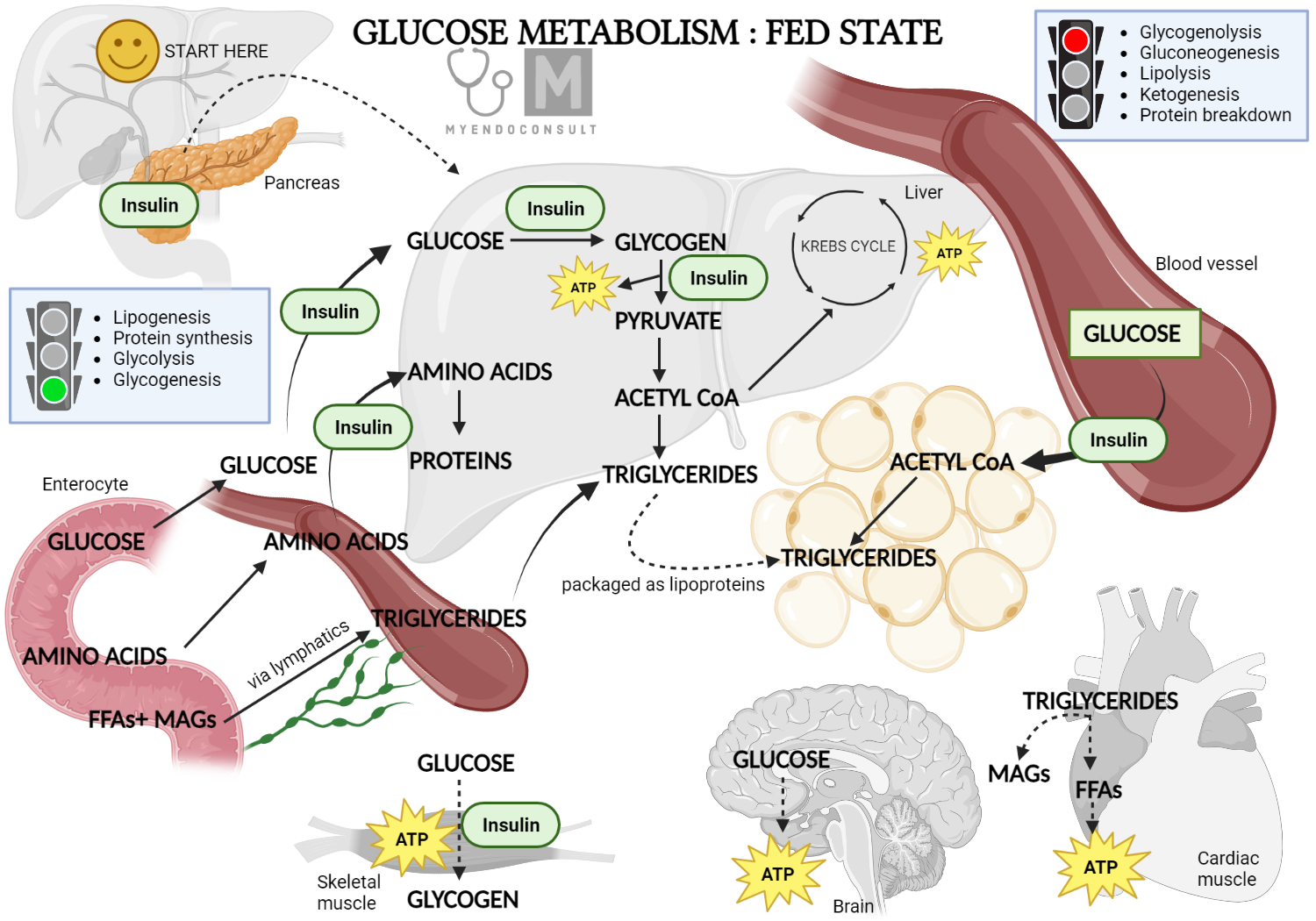
Glucagon: Glucagon has essentially the opposite effect of insulin. It is released when blood glucose levels are low, such as during fasting or intense exercise. Glucagon stimulates gluconeogenesis by upregulating the enzymes involved in this pathway, thereby increasing the production of glucose and restoring blood glucose levels.
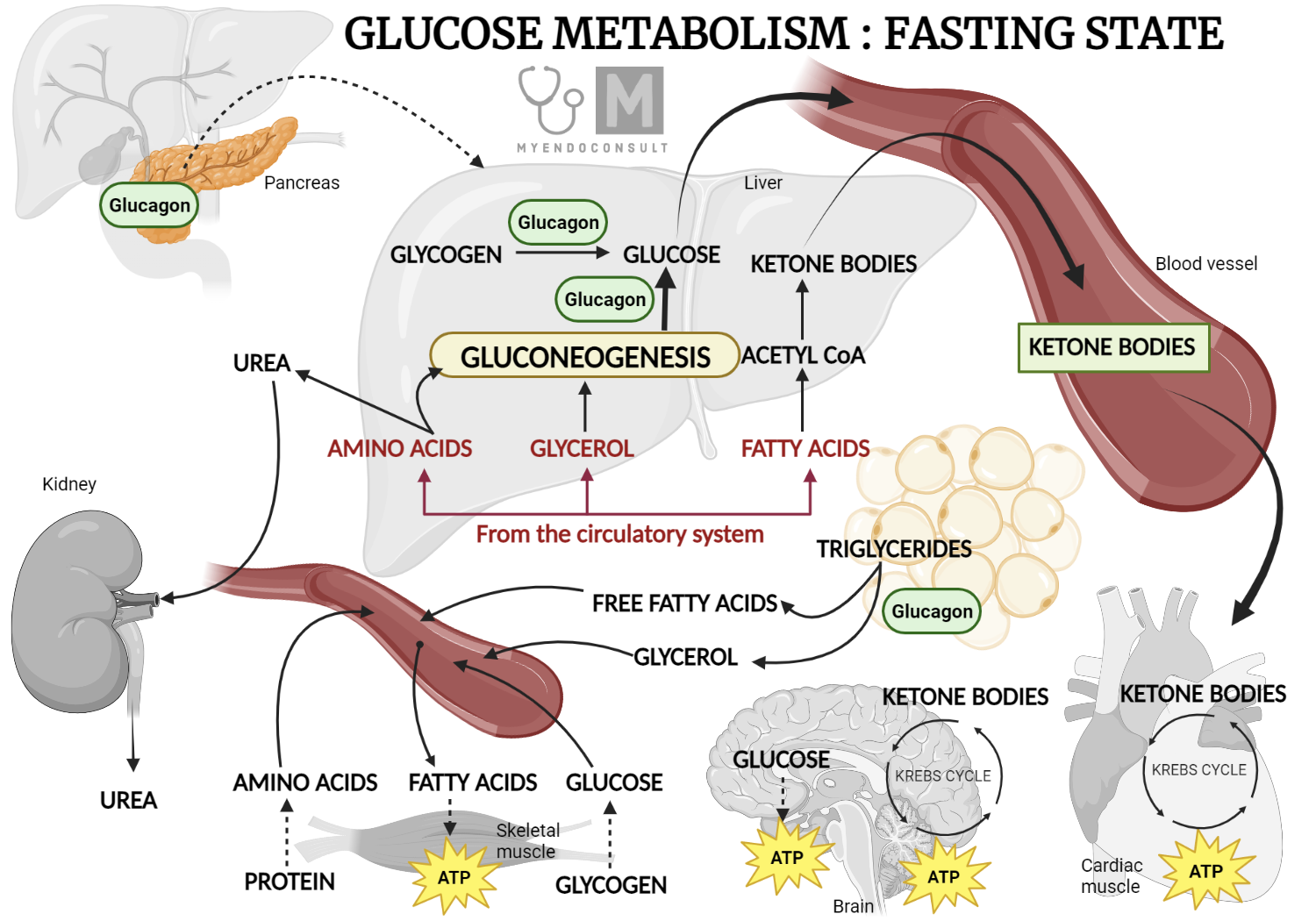
Cortisol: Cortisol, a stress hormone, also plays a role in regulating gluconeogenesis. During periods of stress or fasting, cortisol levels rise, leading to an increase in the rate of gluconeogenesis. Cortisol ensures that adequate glucose is available for the brain and other vital organs, especially during prolonged periods of fasting or stress when glucose from the diet is not available.
In summary, ATP and NADH provide the energy and reducing power necessary for gluconeogenesis, while insulin, glucagon, and cortisol help regulate this pathway according to the body’s metabolic needs and the availability of glucose.
Comparison of Gluconeogenesis and Glycolysis
Gluconeogenesis and glycolysis are two central metabolic pathways with distinct but interconnected roles. They essentially represent reverse processes, with glycolysis breaking down glucose to produce energy and gluconeogenesis synthesizing glucose from non-carbohydrate precursors.
Detailed Comparison of the Two Pathways
Glycolysis is a catabolic pathway that occurs in the cytoplasm of nearly all cells, breaking down one glucose (6-carbon sugar) into two pyruvate molecules (3-carbon compounds). This process releases energy, captured in two molecules of ATP and two molecules of NADH per glucose.
Gluconeogenesis, in contrast, is an anabolic pathway that takes place primarily in the liver (and to a lesser extent, the kidneys). This process consumes energy to generate glucose from non-carbohydrate precursors like lactate, glycerol, and certain amino acids. The newly synthesized glucose can then be released into the bloodstream to maintain glucose homeostasis.
Differences in Steps and Enzymes
Though seven of the steps in gluconeogenesis are the reverse of those in glycolysis, they utilize the same enzymes. However, there are three key steps in glycolysis that are energetically unfavorable to simply reverse. In these cases, gluconeogenesis bypasses these steps using four unique enzymes:
- Pyruvate to PEP: In glycolysis, PEP is converted to pyruvate by pyruvate kinase. Gluconeogenesis bypasses this step by using two enzymes – pyruvate carboxylase and PEP carboxykinase – to convert pyruvate to PEP.
- Fructose-1,6-bisphosphate to Fructose-6-phosphate: This step is catalyzed by fructose-1,6-bisphosphatase in gluconeogenesis, bypassing the reaction in glycolysis where phosphofructokinase-1 converts fructose-6-phosphate to fructose-1,6-bisphosphate.
- Glucose-6-phosphate to Glucose: In gluconeogenesis, glucose-6-phosphatase catalyzes this reaction, bypassing the step in glycolysis where glucokinase or hexokinase converts glucose to glucose-6-phosphate.
Role of Each Pathway in Energy Metabolism
In terms of energy metabolism, glycolysis serves to break down glucose to generate ATP for immediate use by the body, especially in tissues like muscle during exercise. Glycolysis also generates pyruvate, which can be further metabolized in the mitochondria through the citric acid cycle for additional ATP production.
Gluconeogenesis, on the other hand, serves to maintain blood glucose levels during periods of fasting, low carbohydrate intake, or intense exercise. This is crucial as some tissues, such as the brain and red blood cells, primarily rely on glucose for their energy needs. Therefore, gluconeogenesis ensures a steady supply of glucose for these tissues when dietary intake is insufficient.
Role of Gluconeogenesis in Health and Disease
Gluconeogenesis plays a pivotal role in maintaining blood glucose levels, especially when carbohydrate intake is low or absent, such as fasting or intense physical activity. Glucose is the primary energy source for various tissues, including the brain and red blood cells, and gluconeogenesis ensures these tissues have a constant supply of glucose.
Role in Fasting and Starvation
During short-term fasting, liver glycogen stores are the first source of glucose. However, these stores become depleted after about 24 hours. To prevent hypoglycemia, gluconeogenesis in the liver and kidneys starts to generate glucose from non-carbohydrate sources such as lactate, glycerol, and certain amino acids. During prolonged fasting or starvation, gluconeogenesis remains critical, with amino acids from muscle protein breakdown becoming a significant source of glucose production.
Involvement in Diseases such as Diabetes and Metabolic Syndrome
Gluconeogenesis is implicated in several metabolic diseases. In type 2 diabetes and metabolic syndrome, hepatic insulin resistance can lead to excessive glucose production through gluconeogenesis, contributing to hyperglycemia. Meanwhile, in type 1 diabetes, a deficiency of insulin combined with an excess of glucagon can stimulate excessive gluconeogenesis, also resulting in hyperglycemia.
Potential Therapeutic Targets
Given the role of gluconeogenesis in maintaining glucose homeostasis and its dysregulation in diseases, it presents potential therapeutic targets. Drugs that inhibit key gluconeogenic enzymes could potentially help control blood glucose levels in diabetes. For instance, metformin, a first-line drug for type 2 diabetes, is believed to partially exert its glucose-lowering effect by inhibiting hepatic gluconeogenesis.
However, achieving selective inhibition of gluconeogenesis without affecting other metabolic processes is a challenge. Continued research into the precise molecular mechanisms regulating this pathway is necessary to identify more specific and effective therapeutic targets. It’s worth noting that while targeting gluconeogenesis may help manage blood glucose levels in diabetes, it’s essential to address the broader metabolic dysfunctions present in these conditions, including insulin resistance and impaired insulin secretion.
Clinical Case Vignette
Question 1:
A 55-year-old man with a history of type 2 diabetes mellitus is seen in clinic. He has been managing his diabetes with metformin 500mg twice daily and diet control. Despite adherence to his medication regimen and a balanced diet, his fasting blood glucose levels have been consistently elevated at around 200 mg/dL. How does metformin help in reducing this patient’s blood glucose levels?
A. It increases glucose uptake in muscle cells.
B. It stimulates insulin secretion from pancreatic beta cells.
C. It inhibits hepatic gluconeogenesis.
D. It enhances glycogenolysis in the liver.
Answer: C. It inhibits hepatic gluconeogenesis.
Explanation: Metformin is a first-line drug in the treatment of type 2 diabetes and works primarily by inhibiting hepatic gluconeogenesis. This reduces the amount of glucose produced by the liver, helping to maintain blood glucose levels within a normal range. While it can improve insulin sensitivity and therefore potentially increase glucose uptake in muscle (option A), this is not the primary mechanism of metformin’s glucose-lowering effect. Metformin does not stimulate insulin secretion (option B), nor does it enhance glycogenolysis (option D) – it would be counterproductive as both actions would increase blood glucose levels.
Question 2:
A 45-year-old female with a history of adrenal insufficiency has recently been suffering from frequent episodes of hypoglycemia. Her current medications include hydrocortisone 20mg in the morning and 10mg in the afternoon, and fludrocortisone 0.1mg daily. She is otherwise on a normal diet. Which of the following best describes the reason for her hypoglycemic episodes?
A. Reduced glycogen synthesis in the liver.
B. Impaired gluconeogenesis.
C. Enhanced insulin secretion.
D. Increased glycolysis in muscle tissues.
Answer: B. Impaired gluconeogenesis.
Explanation: In adrenal insufficiency, there is a deficiency of cortisol, a hormone that stimulates gluconeogenesis in the liver. When cortisol levels are low, gluconeogenesis is impaired, leading to decreased production of glucose, especially during fasting or between meals. This can result in hypoglycemia, particularly if the patient does not eat regular meals. While options A, C, and D could theoretically lead to hypoglycemia, they are not directly linked to adrenal insufficiency and the patient’s current condition.
Kindly Let Us Know If This Was helpful? Thank You!