Adipose tissue: Anatomy & function
Adipose organ has been classified into brown adipose tissue (BAT) and white adipose tissue (WAT). Brown adipose tissue regulates body temperature by generating heat through the expenditure of stored energy. On the other hand, white adipose tissue serves as energy storage. Energy is stored in white adipose tissue in the form of lipids.
The morphology of white and brown adipose tissues is understandably different. In brown adipocytes, lipids are organized in many droplets (multilocular), while in white adipocytes, they are unilocular (organized in unique droplets) [1]. Another distinguishing feature of the morphology of white and brown adipocytes is the presence of large mitochondria in brown adipocytes. Brown adipocytes are also characterized by the expression of uncoupling protein 1. Uncoupling protein 1 (UCP1) facilitates the uncoupling of oxidative phosphorylation from ATP synthesis ultimately leading to heat production [2].
Initially, brown adipose tissue was thought to be present only in infant humans. However, imaging studies have shown metabolically active brown adipose tissues in the thoracic and supraclavicular regions of adults [3-5].
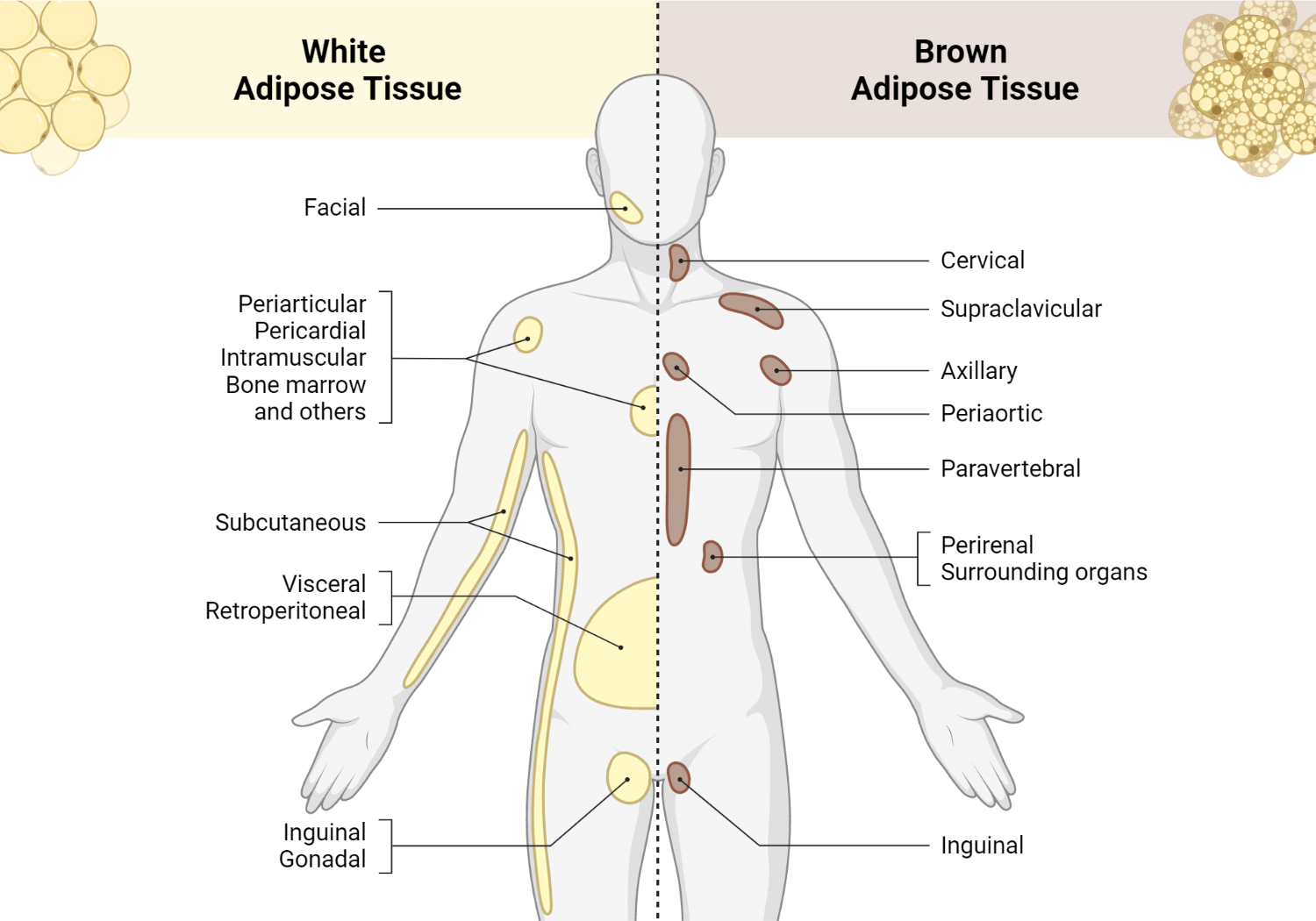
Location of white and brown adipose tissue
The mass and activity of brown adipose tissue is higher in women than in men [5, 6].
It is important to note that the chance of detecting brown adipose tissue activity in humans (irrespective of sex) correlates inversely with age and body mass index [5]. The activity of brown adipose tissue has been observed to be higher in the winter and much reduced in the summer. This may be attributed to the temperature, or more probably to the photoperiod [5, 6]. The activity of brown adipose tissue in healthy humans contributes to the oxidation of whole-body fat as well as diet-induced thermogenesis [7].
Brown and white adipose tissue vary in size, shape, and organelle structure. White adipose tissue has a spherical shape. Each white adipose tissue contains a single lipid droplet that pushes the nucleus and other organelles to the cell’s periphery.
There are multiple lipid droplets in brown adipocytes. These lipid droplets are dispersed throughout an iron-containing mitochondria-enriched cell which gives the cell a brown hue. Brown adipocytes grow between 15 to 50µm. White fat cells have a larger storage capacity and can expand to 100 µm in diameter [8].
Researchers have recently described two additional adipocyte hues – the pink and the beige hues. Beige adipocytes display characteristics of both white and fat cells. Studies have also shown that beige adipocytes develop within white adipocytes [9] or through the trans-differentiation of existing white adipose tissues [10, 11]. However, according to results from gene expression analyses, beige fat cells are typically a distinct form of thermogenic fat cells [9]. They originally formed in rodents that were exposed to cold [12, 13]. Results from several studies have shown that diet [14], exercise [15], prebiotics and postbiotics [16], adipokines, plant-based bioactives, and pharmaceutical agents [17] can induce the browning or beiging of white adipose tissues, which may in turn provide protection against clinical obesity and obesity-related metabolic dysfunction.
The first description of pink adipocytes occurred in 2014, when they were discovered among subcutaneous white adipose tissues of female mice between the 17th and 18th day of pregnancy and persisting throughout the period of lactation.
These fat cells derive from white adipose tissues that assume an epithelial-like feature giving rise to milk-secreting alveoli, and ultimately the tissue’s pink hue [18].
The primary characteristics of pink adipocytes include cytoplasmic projections, compartmentalized lipid droplets, and a large number of organelles including peroxisomes, mitochondria, and rough endoplasmic reticulum.
Adipocyte differentiation and metabolism
The formation of white adipose tissues begins shortly after birth. Mesenchymal stem cells develop into adipoblasts after which they differentiate into preadipocytes. The main phase of adipogenesis involves the proliferation of preadipocytes, which progress into mitosis until they reach growth arrest.
If preadipocytes leave the cell cycle at this point, their morphology changes and accumulate cytoplasmic triglycerides, gaining characteristics of mature adipocytes and loosing the ability to divide [19]. Both brown and white adipocytes originate from the mesoderm, though the precursor cells from which they derive differs.
Mesenchymal stem cells may be committed to a Myf5-positive myogenic lineage (giving rise to brown adipocytes), or a Myf5-negative adipogenic and osteoblatogenic lineage (giving rise to white adipocytes) [20]. Now, even though the adipocytes arise from different lineages, the differentiation of the adipocytes shares the same pathway that involves enhancer-binding/CCAAT proteins [21].
Differentiation in white adipocytes results in the phosphorylation of the cAMP response element-binding protein followed by the induction of the C/EBP-β expression [22]. C/EBP-β binds to DNA, allowing the reentry of preadipocytes into the cell cycle or increasing the levels of peroxisome proliferator-activated receptor-γ (PPAR-γ) or transcription factors C/EBP-α [21]. PPAR-γ plays a key role in the promotion of glucose and lipid metabolism as well as insulin sensitivity of adipocytes.
It is important to note that brown adipose tissues develop before birth because it protects the newborn against cold. Bone morphogenetic protein (BMP)-7 positively controls the differentiation of brown preadipocytes into mature brown adipocytes [23], while myostatin negatively controls the differentiation of brown preadipocytes into mature brown adipocytes [24]. PR domain containing 16 (PRDM16) and C/EBP-β act as transcriptional factors in brown adipocyte differentiation [25]. C/EBP-β is also a primary transcriptional factor that leads to UCP1 expression inducing the process of thermogenesis [26].
The process of transdifferentiation
White adipose depots can potentially switch between energy expenditure and energy storage. As such, in certain physiologic conditions, white adipocytes can transform into brown adipocytes and thus contribute to thermogenic needs [27].
The brite/beige cells in white adipose tissues are derived from precursor cells that are distinct from classical brown adipocytes. These depots have the ability to shift from a white adipose tissue phenotype to a brown adipose tissue-like phenotype, showing gene expression pattern, morphology, and mitochondrial respiratory activity with a close semblance to that of classical brown adipose tissue [28].
The induction of the brown adipose tissue phenotype in white adipose tissue is known as browning response [29]. It is important for clinicians to understand the molecular mechanism of this process since the knowledge of it could be applied in the development of therapeutic strategies to prevent formation of white adipose tissues in excess. Exposure to cold induces the activation of beige/brite cells via different molecular mechanisms. The sympathetic nervous system mediates this conversion through the beta3AR since it has been shown that beta3AR agonist administration can induce the browning response [30].
Transcription factors like PRDM16, C/EBP-beta, PPAR-γ and its coactivator PGC-1α appear to play vital roles in the regulation of the browning program [31]. Thiazolidinediones are used to control glycemia [32]. They are a group of PPAR-γ activator agents that have proven effective in the transformation of white adipose tissues into brown adipose tissue-like tissue in dogs, rats, and mice [33-35]. PGC-1α, a cofactor of PPARγ is inducible by exposure to cold in brown adipose tissue [36].
Also, human white adipose tissue express UCP1 when transfected with PGC-1α. UCP1 is a marker gene of brown adipocytes [37]. PRDM16 and C/EBP-β induce brown phenotype of fat cells. Together, they form a molecular complex that plays an essential role in brown differentiation of mesenchymal stem cells.
Brown adipose tissues and the liver together produce fibroblast growth factor 21 (FGF21). Studies have shown that FGF-21 acts in a paracrine/autocrine manner to boost the expression of UCP1 and other thermogenic genes in fat tissue through the β-klotho receptor [38]. On the other hand, transforming growth factor beta (TGF-beta) has a negative impact on the browning process.
Recent studies have shown that irisin, a hormone produced by skeletal muscles during exercise, induces white-to-brown conversion both in vitro and in vivo [39]. The ability of white adipose tissue to transform itself depending on the need for higher energy consumption is known as plasticity of white adipose tissue. It is worth mentioning that this white adipose tissue plasticity is not solely related to the browning response. Subcutaneous depots of adipose organs may convert into mammary glands during pregnancy and lactation [40]. The lipid content of white adipose tissue is lost during pregnancy, and the white adipocytes acquire epithelial features, forming glandular structures that can produce milk. Part of the epithelial component of the mammary glands converts into adipocytes following lactation, thus allowing the recovery of the adipose component in the subcutaneous depot [40].
The plasticity of adipose cells and their ability to modify their phenotype and adapt to the environmental stimuli satisfies the basic physiological needs of the organism.
Role of adipokines in adipocyte metabolism
There is evidence that molecules secreted by adipose tissue are involved in metabolic homeostasis. There is also considerable evidence that the deficiency of these molecules due to adipocyte dysfunction or massive adiposity may lead to obesity or is related pathologies.
The adipose tissue is therefore considered an endocrine organ, and its actions on target tissues depend on where the fats depots are located. In turn, the location of these fat depots may differ according to its functional state. Having a full understanding of adipose tissue secretome might give some insights into the functions of the body’s most important metabolic regulator.
Leptin is an extremely important and potent adipokine in metabolic regulation. It regulates body weight by sending signals of satiety or appetite to they hypothalamus. The hypothalamus in turn produces and secretes neurotransmitters that adapt energy expenditure and food intake. Leptin suppresses fatty acid synthesis pathway, thus regulating hepatic lipogenesis [41] and improving fatty acid oxidation [42].
Adiponectin is the most secreted adipokine. It enhances sensitivity to insulin and partially reverses insulin resistance in mice [43]. Researchers are currently exploring strategies to boost adiponectin receptor activities or adiponectin levels for the treatment of insulin resistance and obesity-induced inflammation [44].
References
- Cinti S (2001) The adipose organ: morphological perspectives of adipose tissues. Proc Nutr Soc 60:319–328
- Cannon B, Nedergaard J (2004) Brown adipose tissue: function and physiological signifi cance. Physiol Rev 84:277–359
- Nedergaard J, Bengtsson T, Cannon B. Unexpected evidence for active brown adipose tissue in adult humans. Am. J. Physiol. Metab. 2007;293(2):E444–E452
- Zingaretti MC, Crosta F, Vitali A, Guerrieri M, Frontini A, Cannon B, Nedergaard J, Cinti S. The presence of UCP1 demonstrates that metabolically active adipose tissue in the neck of adult humans truly represents brown adipose tissue. FASEB J. 2009;23(9):3113–3120
- Cypess AM, Lehman S, Williams G, Tal I, Rodman D, Goldfine AB, Kuo FC, Palmer EL, Tseng Y-H, Doria A, Kolodny GM, Kahn CR. Identification and importance of brown adipose tissue in adult humans. N. Engl. J. Med. 2009;360(15):1509–1517
- Au-Yong ITH, Thorn N, Ganatra R, Perkins AC, Symonds ME. Brown adipose tissue and seasonal variation in humans. Diabetes. 2009;58(11):2583–7
- Hibi M, Oishi S, Matsushita M, Yoneshiro T, Yamaguchi T, Usui C, Yasunaga K, Katsuragi Y, Kubota K, Tanaka S, Saito M. Brown adipose tissue is involved in diet-induced thermogenesis and whole-body fat utilization in healthy humans. Int. J. Obes. (Lond). 2016;40(11):1655–1661
- Cinti S. The adipose organ at a glance. Dis. Model. Mech. 2012;5(5):588–594
- Wu J, Boström P, Sparks LM, Ye L, Choi JH, Giang A-H, Khandekar M, Virtanen KA, Nuutila P, Schaart G, Huang K, Tu H, van Marken Lichtenbelt WD, Hoeks J, Enerbäck S, Schrauwen P, Spiegelman BM. Beige adipocytes are a distinct type of thermogenic fat cell in mouse and human. Cell. 2012;150(2):366–376
- Barbatelli G, Murano I, Madsen L, Hao Q, Jimenez M, Kristiansen K, Giacobino JP, De Matteis R, Cinti S. The emergence of cold-induced brown adipocytes in mouse white fat depots is determined predominantly by white to brown adipocyte transdifferentiation. Am. J. Physiol. Metab. 2010;298(6):E1244–E1253
- Himms-Hagen J, Melnyk A, Zingaretti MC, Ceresi E, Barbatelli G, Cinti S. Multilocular fat cells in WAT of CL-316243-treated rats derive directly from white adipocytes. Am. J. Physiol. Physiol. 2000;279(3):C670–C681.
- Young P, Arch JRS, Ashwell M. Brown adipose tissue in the parametrial fat pad of the mouse. FEBS Lett. 1984;167(1):10–14
- Loncar D, Afzelius BA, Cannon B. Epididymal white adipose tissue after cold stress in rats. I. Nonmitochondrial changes. J. Ultrastruct. Mol. Struct. Res. 1988;101(2–3):109–122.
- Wang S, Pan M-H, Hung W-L, Tung Y-C, Ho C-T. From white to beige adipocytes: therapeutic potential of dietary molecules against obesity and their molecular mechanisms. Food Funct. 2019;10:1263–1279
- Mika A, Macaluso F, Barone R, Di Felice V, Sledzinski T. Effect of exercise on fatty acid metabolism and adipokine secretion in adipose tissue. Front. Physiol. 2019;10(26):1–7.
- Reynés B, Palou M, Rodríguez AM, Palou A. Regulation of adaptive thermogenesis and browning by prebiotics and postbiotics. Front. Physiol. 2018;9:1–15
- Kaisanlahti A, Glumoff T. Browning of white fat: agents and implications for beige adipose tissue to type 2 diabetes. J. Physiol. Biochem. 2019;75(1):1–10
- Giordano A, Smorlesi A, Frontini A, Barbatelli G, Cinti S. MECHANISMS IN ENDOCRINOLOGY: White, brown and pink adipocytes: the extraordinary plasticity of the adipose organ. Eur. J. Endocrinol. 2014;170(5):R159–R171
- Otto TC, Lane MD (2005) Adipose development: from stem cell to adipocyte. Crit Rev Biochem Mol Biol 40:229–242
- Timmons JA, Wennmalm K, Larsson O, Walden TB, Lassmann T, Petrovic N, Hamilton DL, Gimeno RE, Wahlestedt C, Baar K et al (2007) Myogenic gene expression signature establishes that brown and white adipocytes originate from distinct cell lineages. Proc Natl Acad Sci U S A 104:4401–4406
- Rosen ED, MacDougald OA (2006) Adipocyte differentiation from the inside out. Nat Rev Mol Cell Biol 7:885–896
- Zhang JW, Tang QQ, Vinson C, Lane MD (2004) Dominant-negative C/EBP disrupts mitotic clonal expansion and differentiation of 3T3-L1 preadipocytes. Proc Natl Acad Sci U S A 101:43–47
- Tseng YH, Kokkotou E, Schulz TJ, Huang TL, Winnay JN, Taniguchi CM, Tran TT, Suzuki R, Espinoza DO, Yamamoto Y et al (2008) New role of bone morphogenetic protein 7 in brown adipogenesis and energy expenditure. Nature 454:1000–1004
- Kim WK, Choi HR, Park SG, Ko Y, Bae KH, Lee SC (2012) Myostatin inhibits brown adipocyte differentiation via regulation of Smad3-mediated β-catenin stabilization. Int J Biochem Cell Biol 44:327–334
- Seale P, Bjork B, Yang W, Kajimura S, Chin S, Kuang S, Scimè A, Devarakonda S, Conroe HM, Erdjument-Bromage H et al (2008) PRDM16 controls a brown fat/skeletal muscle switch. Nature 454:961–967
- Kajimura S, Seale P, Kubota K, Lunsford E, Frangioni JV, Gygi SP, Spiegelman BM (2009) Initiation of myoblast to brown fat switch by a PRDM16-C/EBP-beta transcriptional complex. Nature 460:1154–1158
- Cinti S (2009) Transdifferentiation properties of adipocytes in the adipose organ. Am J Physiol Endocrinol Metab 297:E977
- Wu J, Cohen P, Spiegelman BM (2013) Adaptive thermogenesis in adipocytes: is beige the new brown. Genes Dev 27:234–250
- Petrovic N, Walden TB, Shabalina IG, Timmons JA, Cannon B, Nedergaard J (2010) Chronic peroxisome proliferator-activated receptor gamma (PPARgamma) activation of epididymally derived white adipocyte cultures reveals a population of thermogenically competent, UCP1- containing adipocytes molecularly distinct from classic brown adipocytes. J Biol Chem 285:7153–7164
- Himms-Hagen J, Melnyk A, Zingaretti MC, Ceresi E, Barbatelli G, Cinti S (2000) Multilocular fat cells in WAT of CL-316243-treated rats derive directly from white adipocytes. Am J Physiol Cell Physiol 279:C670–C681
- . Kajimura S, Seale P, Spiegelman BM (2010) Transcriptional control of brown fat development. Cell Metab 11:257–262
- Schoonjans K, Auwerx J (2000) Thiazolidinediones: an update. Lancet 355:1008–1010
- Toseland CD, Campbell S, Francis I, Bugelski PJ, Mehdi N (2001) Comparison of adipose tissue changes following administration of rosiglitazone in the dog and rat. Diabetes Obes Metab 3:163–170
- Wilson-Fritch L, Nicoloro S, Chouinard M et al (2004) Mitochondrial remodeling in adipose tissue associated with obesity and treatment with rosiglitazone. J Clin Invest 114:1281–1289
- Koh YJ, Park BH, Park JH et al (2009) Activation of PPAR gamma induces profound multilocularization of adipocytes in adult mouse white adipose tissues. Exp Mol Med 41:880–895
- Puigserver P, Spiegelman BM (2003) Peroxisome proliferator-activated receptor-gamma coactivator 1 alpha (PGC-1 alpha): transcriptional coactivator and metabolic regulator. Endocr Rev 24:78–90
- Tiraby C, Tavernier G, Lefort C et al (2003) Acquirement of brown fat cell features by human white adipocytes. J Biol Chem 278:33370–33376
- Hondares E, Rosell M, Gonzalez FJ, Giralt M, Iglesias R, Villarroya F (2010) Hepatic FGF21 expression is induced at birth via PPARalpha in response to milk intake and contributes to thermogenic activation of neonatal brown fat. Cell Metab 11:206–212
- Bostrom P, Wu J, Jedrychowski MP et al (2012) A PGC1-alpha-dependent myokine that drives brown-fat-like development of white fat and thermogenesis. Nature 481:463–468
- Giordano A, Smorlesi A, Frontini A, Barbatelli G, Cinti S (2014) White, brown and pink adipocytes: the extraordinary plasticity of the adipose organ. Eur J Endocrinol 170(5):R159–71
- Cohen P, Miyazaki M, Socci ND, Hagge-Greenberg A, Liedtke W, Soukas AA, Sharma R, Hudgins LC, Ntambi JM, Friedman JM (2002) Role for stearoyl-CoA desaturase-1 in leptinmediated weight loss. Science 297:240–243
- Minokoshi Y, Kim YB, Peroni OD, Fryer LG, Muller C, Carling D, Kahn BB (2002) Leptin stimulates fatty-acid oxidation by activating AMP-activated protein kinase. Nature 415:339–343
- Berg AH, Combs TP, Du X, Brownlee M, Scherer PE (2001) The adipocyte-secreted protein Acrp30 enhances hepatic insulin action. Nat Med 7:947–953
- Yamauchi T, Kadowaki T (2008) Physiological and pathophysiological roles of adiponectin and adiponectin receptors in the integrated regulation of metabolic and cardiovascular diseases. Int J Obes 32(Suppl 7):S13–S18
Kindly Let Us Know If This Was helpful? Thank You!