Glycolysis is a fundamental pathway in cellular metabolism, converting glucose into pyruvate in a series of enzymatically catalyzed reactions. Occurring in the cytosol of nearly all cells, glycolysis is the initial stage of both aerobic and anaerobic respiration. This article offers a detailed examination of glycolysis, highlighting its mechanism, regulation, and significance in cellular energy metabolism.
Mechanism of Glycolysis
Glycolysis is a ten-step process that can be divided into two phases: the preparatory (or investment) phase and the payoff phase. Each phase comprises five steps:
The Preparatory Phase
- Glucose to Glucose-6-Phosphate (G6P): The enzyme hexokinase catalyzes the phosphorylation of glucose using a molecule of ATP. The resulting G6P is more reactive and trapped within the cell.
- G6P to Fructose-6-Phosphate (F6P): The enzyme phosphoglucose isomerase rearranges G6P into F6P.
- F6P to Fructose-1,6-Bisphosphate (F1,6BP): The enzyme phosphofructokinase-1 (PFK-1) adds another phosphate group to F6P, creating F1,6BP. This step, another ATP investment, is a critical regulatory point in glycolysis.
- F1,6BP to Dihydroxyacetone Phosphate (DHAP) and Glyceraldehyde-3-Phosphate (G3P): The enzyme aldolase splits F1,6BP into two three-carbon molecules: DHAP and G3P.
- DHAP to G3P: The enzyme triose phosphate isomerase converts DHAP into another G3P molecule. At this point, each glucose molecule is broken down into two G3P molecules, and the second phase of glycolysis begins.
The Payoff Phase
- G3P to 1,3-Bisphosphoglycerate (1,3BPG): The enzyme glyceraldehyde-3-phosphate dehydrogenase catalyzes the oxidation of G3P into 1,3BPG, producing NADH in the process.
- 1,3BPG to 3-Phosphoglycerate (3PG): The enzyme phosphoglycerate kinase generates ATP by substrate-level phosphorylation while converting 1,3BPG into 3PG.
- 3PG to 2-Phosphoglycerate (2PG): The enzyme phosphoglycerate mutase relocates the phosphate group from the third carbon to the second carbon, creating 2PG.
- 2PG to Phosphoenolpyruvate (PEP): The enzyme enolase catalyzes the dehydration of 2PG, generating PEP.
- PEP to Pyruvate: The enzyme pyruvate kinase generates another molecule of ATP by substrate-level phosphorylation and converts PEP into pyruvate.
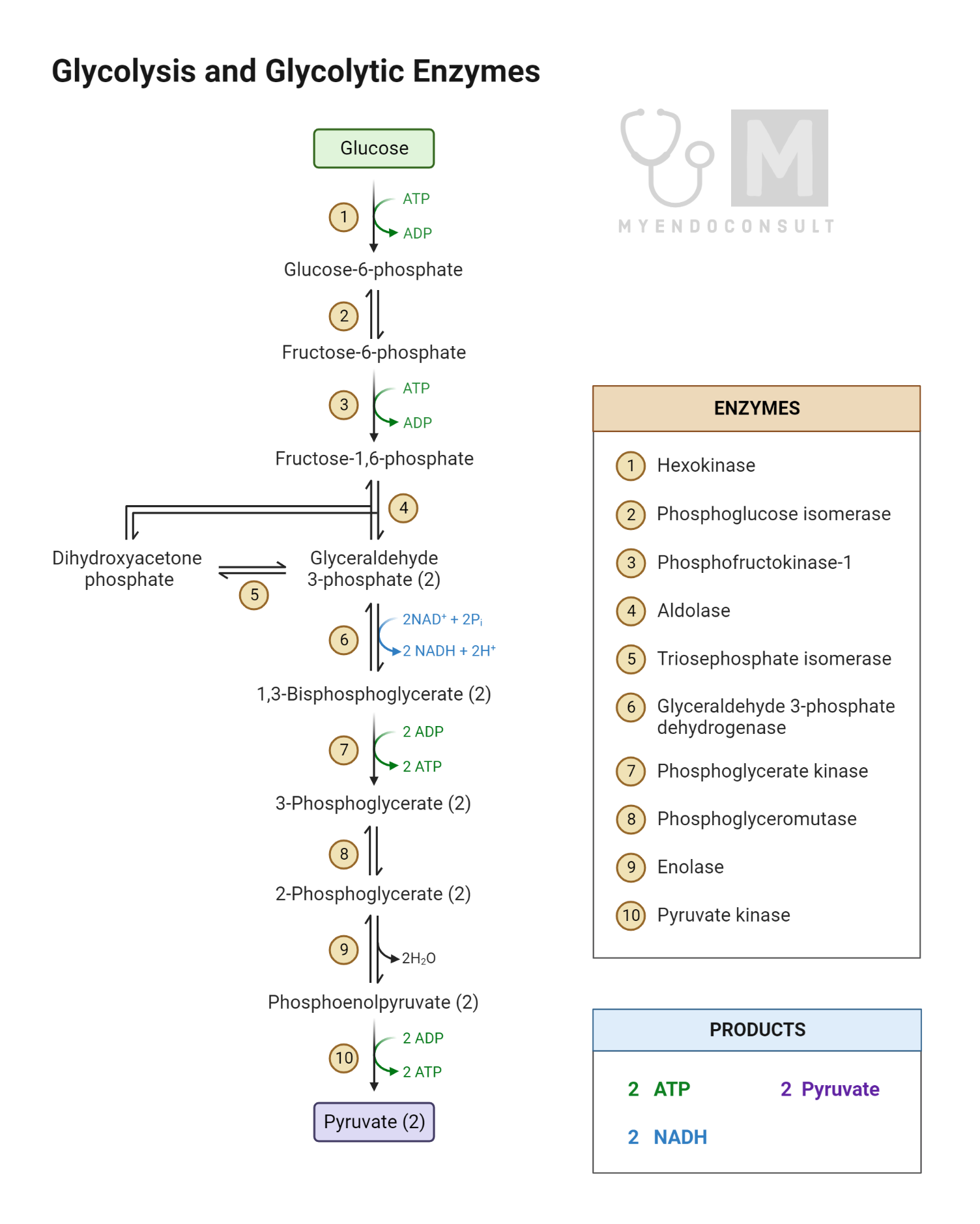
Overall, the glycolysis pathway produces a net gain of two ATP molecules and two NADH molecules per glucose molecule. The end product, pyruvate, can be further processed under aerobic conditions via the TCA cycle or converted to lactate or ethanol under anaerobic conditions.
Regulation of Glycolysis
The regulation of glycolysis is primarily focused on the three irreversible steps catalyzed by hexokinase, phosphofructokinase-1 (PFK-1), and pyruvate kinase. These enzymes are allosterically regulated to ensure that glycolysis matches the energy needs of the cell.
- Hexokinase: This enzyme is inhibited by its product, glucose-6-phosphate (G6P), when G6P levels are high. This is an example of feedback inhibition, preventing the unnecessary expenditure of ATP when there is already ample G6P for glycolysis or other metabolic pathways.
- Phosphofructokinase-1 (PFK-1): This enzyme is the primary control point of glycolysis. When ATP levels are high, ATP binds to an allosteric site on PFK-1, reducing its activity and slowing glycolysis. Conversely, an increase in ADP levels, indicating a high demand for ATP, promotes PFK-1 activity. This enzyme is also stimulated by fructose 2,6-bisphosphate (F2,6BP), a potent activator of glycolysis.
- Pyruvate Kinase: This enzyme is also inhibited by high ATP levels, slowing the final step of glycolysis when the energy demand is low. It’s also positively regulated by fructose 1,6-bisphosphate (F1,6BP), a mechanism called feed-forward stimulation.
Importance of Glycolysis
Glycolysis is a central metabolic pathway, serving several crucial functions in the cell:
- Energy Production: Glycolysis is the initial stage of glucose oxidation, providing ATP rapidly, albeit less efficiently than oxidative phosphorylation. In conditions of low oxygen, such as intense exercise or in certain tissues, glycolysis followed by fermentation can provide ATP anaerobically.
- Intermediates for Biosynthesis: Intermediates from glycolysis serve as precursors for several biosynthetic pathways. For example, glucose-6-phosphate can be channeled into the pentose phosphate pathway for nucleotide synthesis, and 3-phosphoglycerate can be converted into serine and other amino acids.
- NADH Production: Glycolysis generates NADH, which can be used to generate further ATP via oxidative phosphorylation under aerobic conditions, or regenerate NAD+ under anaerobic conditions, allowing glycolysis to continue.
Aberrant glycolysis is implicated in several disease states, notably cancer. The Warburg effect, where cancer cells prefer glycolysis over oxidative phosphorylation for energy production, is a target of ongoing research. Understanding the complexities of glycolysis and its regulation could pave the way for innovative therapies for these conditions.
Comparison of Glycolysis and Gluconeogenesis
Glycolysis and gluconeogenesis are two critical metabolic pathways with opposing roles in glucose metabolism. While glycolysis breaks down glucose to generate energy, gluconeogenesis synthesizes glucose from non-carbohydrate precursors. Here’s a comparison of these two pathways:
Process
- Glycolysis is a catabolic pathway that breaks down a molecule of glucose into two molecules of pyruvate, yielding a net gain of two molecules of ATP and two NADH. This process takes place in the cytoplasm of the cell.
- Gluconeogenesis is an anabolic pathway that synthesizes glucose from non-carbohydrate precursors like lactate, glycerol, or certain amino acids. This process primarily occurs in the liver and, to a lesser extent, in the kidneys.
Energy
- Glycolysis generates energy in the form of ATP and NADH, which can be used for additional ATP production via the electron transport chain.
- Gluconeogenesis consumes ATP. It’s an energy-demanding process because it works against the concentration gradient, synthesizing glucose when glucose levels are low.
Regulation
- Both pathways are subject to complex regulation to ensure glucose homeostasis. They are typically reciprocal, meaning when one pathway is activated, the other is inhibited.
- Glycolysis is stimulated when the energy charge of the cell is low, indicated by high levels of AMP and ADP. Conversely, when energy levels are high (high ATP, low AMP), glycolysis is inhibited. The critical regulatory step is the conversion of fructose-6-phosphate to fructose-1,6-bisphosphate by phosphofructokinase-1 (PFK-1).
- Gluconeogenesis is activated during fasting, prolonged exercise, or times of low carbohydrate intake when the body needs to maintain blood glucose levels. The key regulatory points are the reactions that bypass the irreversible steps of glycolysis, specifically catalyzed by the enzymes pyruvate carboxylase, phosphoenolpyruvate carboxykinase (PEPCK), fructose-1,6-bisphosphatase, and glucose-6-phosphatase.
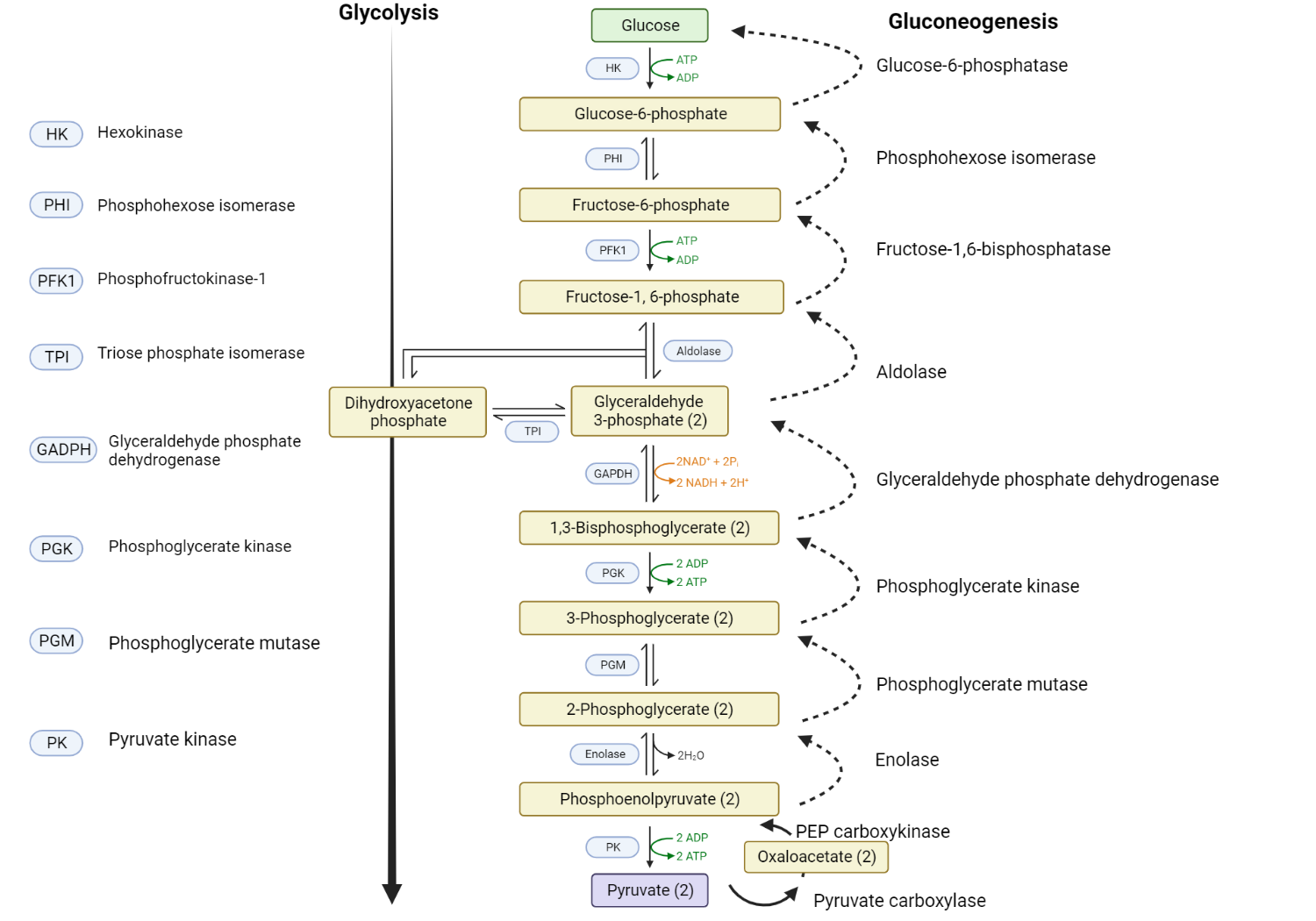
Role in Disease
- Aberrant regulation of glycolysis is seen in cancer cells, which tend to favor glycolysis over oxidative phosphorylation for energy production, even in the presence of oxygen, a phenomenon known as the Warburg effect.
- Defects in the regulation of gluconeogenesis can contribute to hyperglycemia observed in type 2 diabetes.
In summary, glycolysis and gluconeogenesis are opposing but interconnected pathways in glucose metabolism. Understanding their interplay is essential for understanding and treating metabolic diseases.
Conclusion
Glycolysis, as a foundational process in cellular metabolism, is fundamental to life. It serves as the gatekeeper for the catabolic and anabolic pathways of glucose metabolism, determining the route glucose takes based on the energy demands of the cell. Its ten-step process, regulated by key enzymes, ensures that the pathway can adapt to the constantly changing needs of the cell and the organism as a whole.
Beyond serving as a source of energy in the form of ATP and NADH, glycolysis also supplies crucial intermediates for a range of other metabolic pathways. The flexibility of glycolysis and its central role in metabolism makes it a key area of interest in understanding health and disease. Aberrations in glycolysis have been implicated in a variety of disease states, from cancer to metabolic disorders.
Indeed, the study of glycolysis has the potential to continue unlocking therapeutic targets for a range of diseases. Despite being discovered over a century ago, new layers of complexity and regulation in glycolysis are still being unveiled, attesting to the depth and intricacy of our cellular metabolic processes. As our understanding of glycolysis deepens, we not only gain insight into the most basic processes of life, but also novel approaches for therapeutic intervention in disease.
Kindly Let Us Know If This Was helpful? Thank You!