Introduction
In 1849, Claude Bernard introduced the concept of homeostasis, positing that the internal cellular environment (milieu interieur) is under constant regulation. His work in 1855, demonstrating the hepatic synthesis and release of glucose, further extended this concept, underscoring internal biochemical processes crucial for understanding endocrinology. While glucose is not classified as a hormone today, Bernard’s contributions significantly advanced the field.
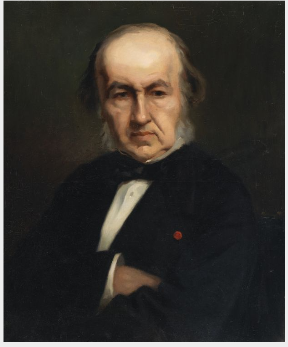
Historically, endocrine glands have been referenced for millennia, but their formal scientific classification as such emerged only in the early 20th century. This period marked the adoption of methodologies to validate endocrine functions in tissues and organs. Given that hormones are secreted into the bloodstream, endocrine glands characteristically exhibit higher blood flow per tissue mass than other organs. Advancements in assay techniques for hormone quantification in blood were pivotal in further elucidating endocrine physiology. These assays allowed for comparative analysis of hormone concentrations in venous outflow versus arterial inflow from endocrine glands, aiding in the localization of endocrine pathologies, including tumors.
The experimental approach to understanding endocrine function, as exemplified by Berthold’s 1849 study on testes and the phenotypic changes in cockerels, was foundational. This and similar studies, where the removal and transplantation of endocrine glands led to observable physiological alterations, were critical in identifying classical endocrine glands like the thyroid, parathyroids, gonads, and adrenals.
Modern endocrinology acknowledges a broader spectrum of hormone-producing tissues beyond the classical endocrine glands. Organs such as the heart, lungs, kidneys, and liver, traditionally recognized for other physiological functions, are now understood to also have hormonal roles. This expanding view challenges the traditional paradigm of endocrine functionality and underscores the complexity of inter-organ communication and regulation in human physiology. The surgical removal of these multi-functional organs for endocrine study poses obvious challenges, necessitating alternative investigative approaches.
What is an endocrine gland?
An endocrine gland, or tissue, is essentially a cluster of cells that produce a chemical (hormone) and release it directly into the bloodstream. These glands are typically identified by their specialized cellular structures that facilitate the secretion of hormones. In contrast, exocrine glands like salivary glands or parts of the pancreas release their products into ducts that exit the body.
While traditionally, certain glands were easily classified as endocrine based on this definition, modern understanding includes a wider range of glands and tissues. These ‘classic’ endocrine glands include the gonads, thyroid, adrenals, parathyroids, pancreatic islets of Langerhans, and the pituitary, each producing one or more types of hormones, specifically those derived from amino acids.
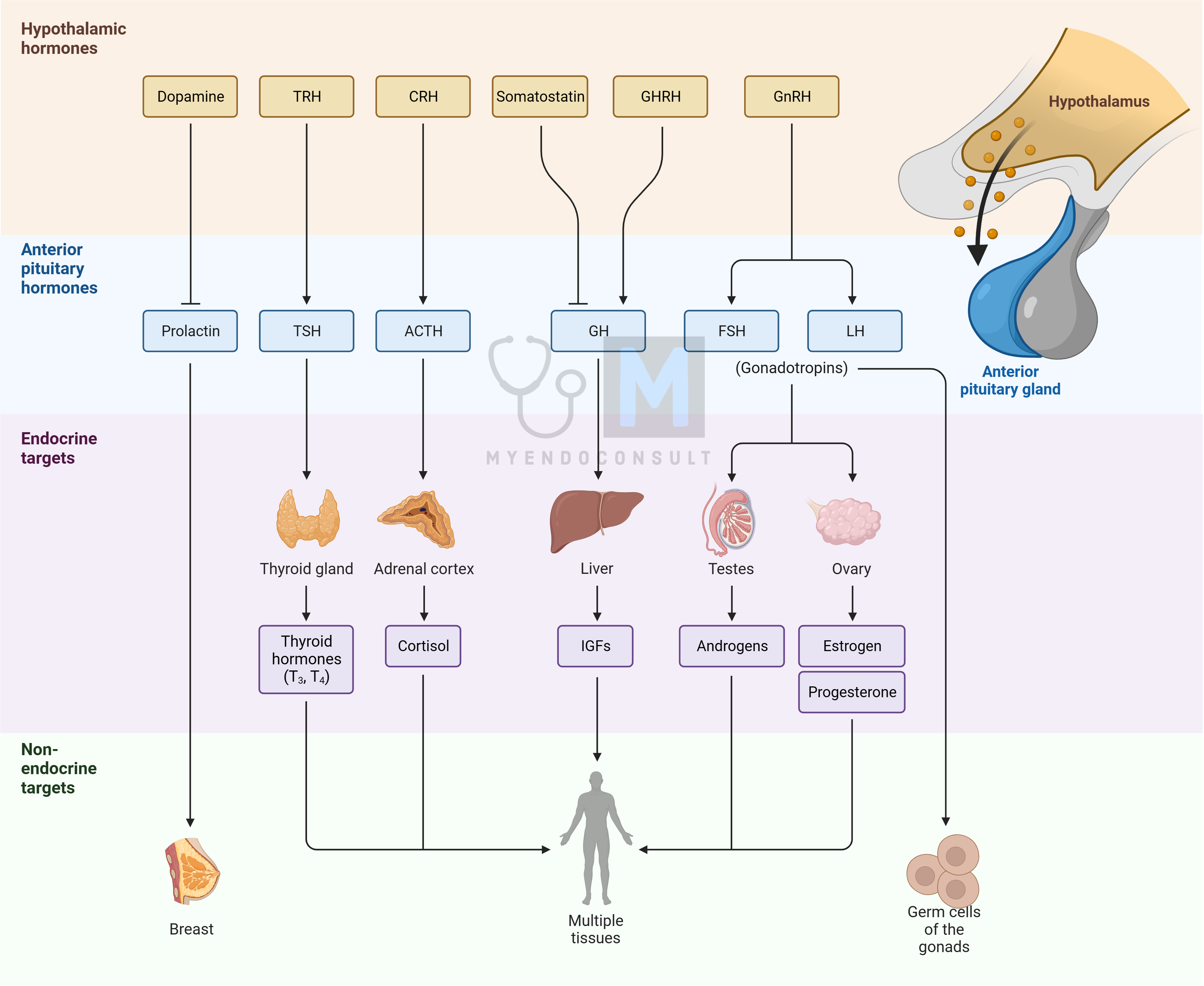
Another significant source of hormones is the gastrointestinal tract. The term “hormone” was first used in 1909 by physiologists William Bayliss and Ernest Starling to describe secretin, a hormone produced by the gastrointestinal tract. This organ, known primarily for its role in digestion and absorption, also produces numerous hormones. Many of these hormones have gastrointestinal effects and are of interest to gastroenterologists. However, some have broader impacts, including on the central nervous system (CNS), making them a focal point for endocrinologists studying the regulatory mechanisms of food intake, hunger, and appetite.
The central nervous system, particularly the hypothalamus, plays a crucial role in endocrine functions. The hypothalamus releases molecules into a specific blood portal system connected to the anterior pituitary or directly into the general circulation through the posterior pituitary. Beyond the hypothalamus, other brain regions may secrete molecules into brain fluids like the intracerebroventricular fluid or general brain extravascular fluids, facilitating communication within the brain.
An interesting aspect of brain endocrinology is the pineal gland, often referred to in Hinduism and Buddhism as the third or inner eye, symbolizing enlightenment. This gland produces melatonin, a hormone that regulates various functions linked to the body’s internal circadian clock located in the suprachiasmatic nucleus of the hypothalamus. Melatonin production peaks at night and is inhibited by daylight.
The placenta is another significant endocrine tissue, especially during pregnancy. It produces many hormones, often in conjunction with the developing fetus, forming what is known as the feto-placental unit. These hormones play vital roles in reproductive functions.
In recent times, it has been discovered that organs traditionally known for other physiological functions also have endocrine roles. These include the liver, kidneys, heart, blood, adipose tissue, and immune tissues like lymphoid tissue, which produce molecules with endocrine effects on distant target cells.
Endocrine glands are dynamic, responding to various signals that can be either stimulatory or inhibitory. This complexity allows for the intricate regulation of hormone production and release into the general circulation, highlighting the integrated nature of endocrine system signaling.
What is a hormone?
What exactly is a hormone? It’s a molecule created by certain cells (in an endocrine gland or tissue) that is released from these cells and travels to its target cells, usually through the blood. Hormones prompt a specific reaction in their target cells, typically for the organism’s benefit. This is part of the body’s way of maintaining balance in response to changes in the internal or external environment. Think of hormones as messenger molecules, relaying signals from one part of the body to another. The way target cells respond to a hormone often involves a series of internal cellular reactions, which could be called ‘second’ messenger systems.
However, not every molecule produced by the body fits the definition of a hormone. For instance, substances like nutrients or waste products don’t qualify. Claude Bernard discovered that the liver releases glucose, an energy substrate, but this doesn’t make it a hormone. Nonetheless, the liver does produce other molecules that are not just nutrients or waste but act as true messengers (‘first’ messengers) in the bloodstream, making it an endocrine tissue.
It’s also important to distinguish hormones from neurotransmitters or neuromodulators, which are chemicals released from nerve terminals and act in the tiny gaps between neurons. However, this doesn’t rule out that neurons, particularly in the central nervous system, can function as endocrine cells, producing hormones. This intersection of neuron and hormone study is a growing field known as neuroendocrinology.
The classification of a molecule as a hormone can sometimes be challenging. Take cytokines, for example. These are proteins made by immune cells that act as communicators between cells, transported by blood, and can affect various body parts, including the CNS. This makes them function similarly to hormones.
To figure out if a molecule is a hormone, one method is to check if it has specific receptors on its target cells. This helps distinguish hormones from simple nutrients and waste products, which don’t have such receptors. Sometimes, the discovery of a new protein, which may be a receptor, leads to identifying a new hormone. Until the hormone (or ligand) that binds to this ‘orphan receptor’ is found, the receptor remains unpaired.
Hormones are special chemicals released by certain cells into the bloodstream to act on distant cells. However, they might also enter other body fluids like cerebrospinal fluid, seminal fluid, amniotic fluid, and lymph. These fluids contain various substances, including water, solutes, ions, cells, and cell fragments. Hormones can be hydrophilic (water-loving) like amino acids, peptides, and proteins, or lipophilic (fat-loving) like steroids. The chemical nature of a hormone significantly influences its synthesis, storage, release, transport, and action mechanism.
Interestingly, some gases also function as hormones, like nitric oxide, which is produced in various tissues and acts locally due to its short lifespan in circulation.
Most hormones work on cells far from where they are produced, but some, like nitric oxide, affect nearby cells, a process known as paracrine effect. Additionally, some hormones influence the cells that produce them, impacting their own synthesis, storage, and release, known as autocrine effect.
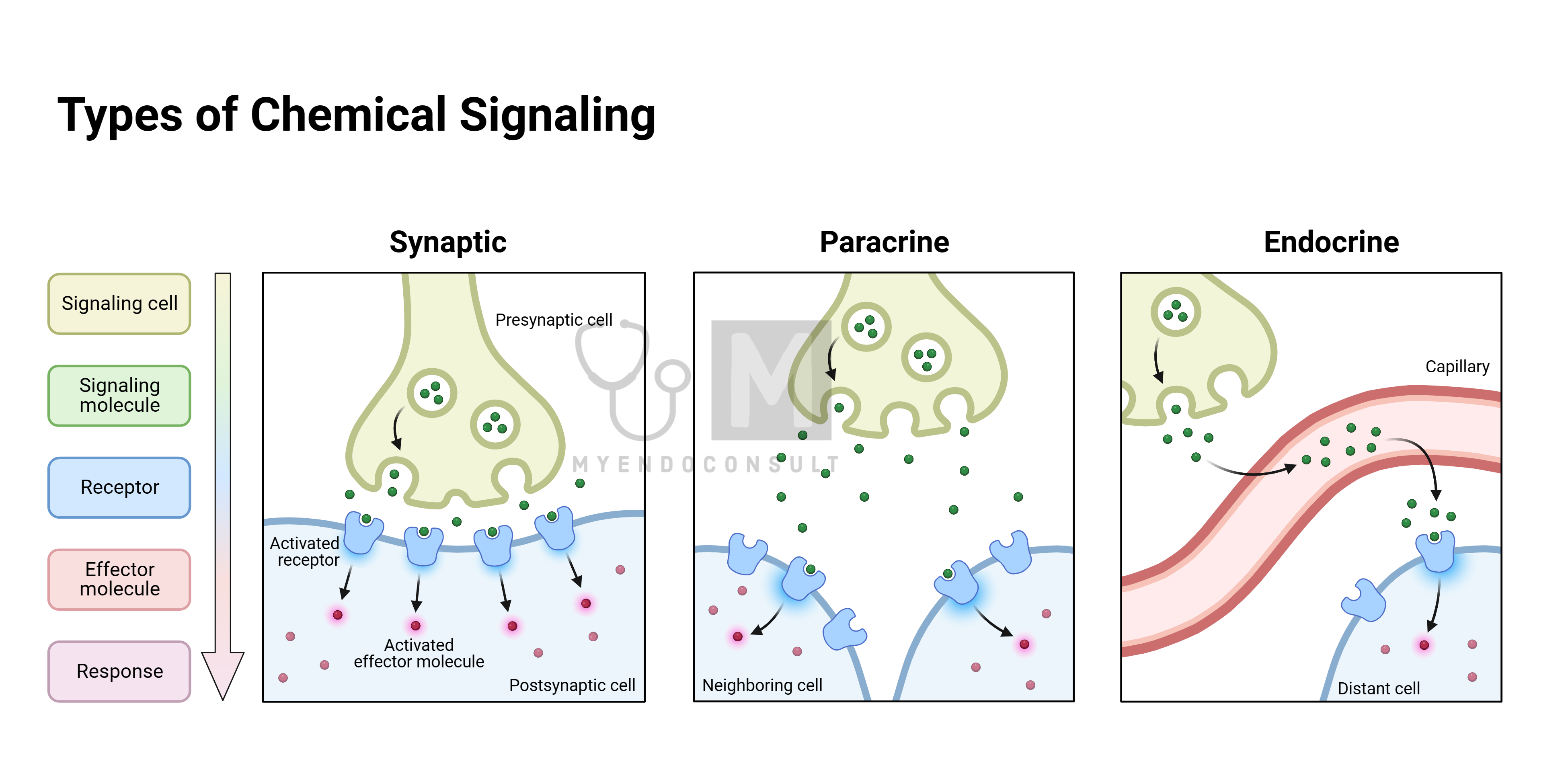
Another concept is the cryptocrine action, where a hormone acts within a confined space close to its producing cell. An example is the Sertoli cell in the testis, producing factors that affect developing spermatids within its enclosed environment.
Adjacent cells often have unique physical connections that allow them to interact closely. One way they do this is through gap junctions, where the cell membranes are in direct contact. These junctions contain membrane proteins known as connexins, which form pores (connexons) that let small molecules like ions and nutrients pass from one cell to another.
Another type of cell interaction involves the formation of tight junctions, which temporarily bind adjacent cells together. These junctions are made of transmembrane proteins and can trap extracellular fluid between the cells. When a cell secretes a molecule into this small space, it can reach a high concentration locally. If the neighboring cell has receptors for this molecule, it can have a specific effect that wouldn’t happen if the molecule were released into the general circulation, where it would be more diluted.
The main hormones, their chemical types, and their primary production sites are listed in a table (not included here). It’s worth noting that many hormones and some endocrine glands are known by multiple names, which are used interchangeably.
Comparison of hormones and transmitters of the nervous system
Hormones and neurotransmitters are both crucial chemical messengers in the body, but they function in distinct ways. Hormones are part of a regulatory system that maintains the body’s balance (homeostasis) in response to internal and external changes. The nervous system, on the other hand, consists of neurons that communicate within the central nervous system (CNS) or with target cells like muscle fibers through synapses or neuromuscular junctions.
Neurons produce neurosecretions (neurotransmitters) that are typically released across synaptic gaps or at neuromuscular junctions. However, when these neurosecretions are released into the blood, they function as hormones. Some neurosecretory molecules can also be transported to distant target cells via cerebrospinal fluid or brain extracellular fluid, qualifying them as hormones.
The hypothalamus, a critical part of the brain for regulating internal environments, influences peripheral endocrine glands. It releases neurosecretions into a specialized blood system that targets the pituitary gland, integrating the hypothalamus into both the CNS and endocrine system. This hypothalamo-pituitary axis is a key focus of endocrine regulation and is often likened to an orchestra conductor (hypothalamus) leading the first violin (pituitary gland).
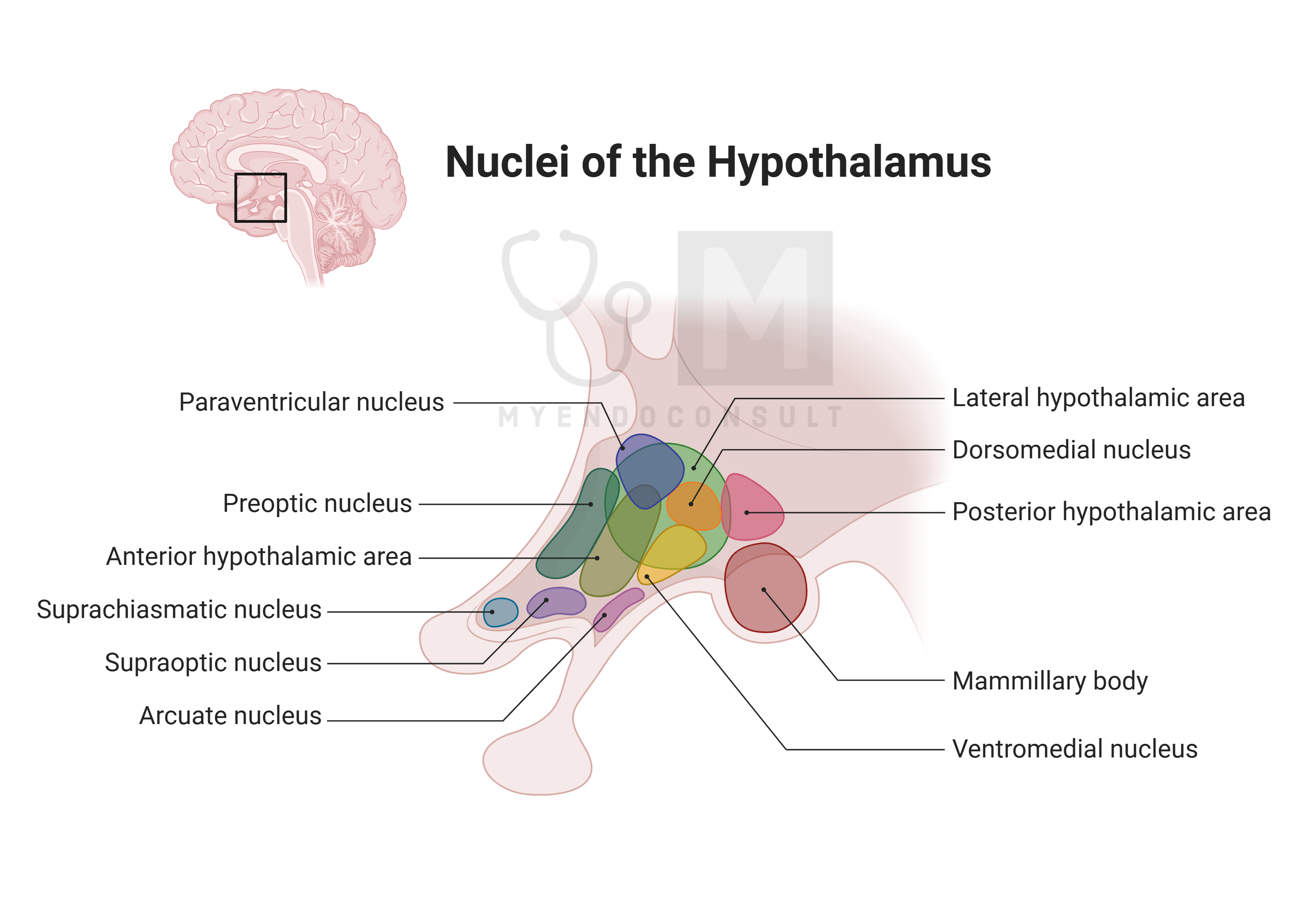
Both neural and endocrine systems rely on specific chemicals as neurotransmitters or hormones, but they have distinct characteristics:
- Neurotransmitter Composition: Traditionally, neurotransmitters are small molecules like acetylcholine or amino acids. Hormones can be amino acid-derived but also include polypeptides, steroids, and larger proteins. The line blurs as neurons can also produce polypeptides and neurosteroids, and some hormones thought to be exclusive to the periphery are also found in the CNS.
- Release Mechanisms: Both neurotransmitters and hormones are often released from vesicles into the surrounding fluid through similar processes involving calcium ions and exocytosis, utilizing microtubules and filaments.
- Action Mechanisms: They generally act through similar mechanisms, involving ion channels or G protein–related receptors.
Despite these similarities, there are key differences. Neurotransmitters typically act in the immediate vicinity of their release, influencing adjacent or postsynaptic neurons rapidly. Hormones, on the other hand, often have a broader and more prolonged impact, affecting distant cells throughout the body. The distinction between neurotransmitters and hormones is therefore not just in their composition and production, but also in their range and duration of action.
Table. 1. Comparison of hormones and neurotransmitters
Feature | Neurotransmitter | Hormone |
Site of action | Specific neurons | Non-neuronal tissue |
Mode of transfer | Across synaptic clefts of neurons | Blood or other fluid in the extracellular space |
Onset of action | A few milliseconds (rapid onset) | Seconds up to days depending on the hormone |
Hormone Synthesis Process
Cells in the body synthesize various molecules essential for their proper functioning. Some of these molecules, like enzymes, remain inside the cell, while others, such as glucose, are exported to other parts of the body. Intracellular molecules include carbohydrates (used for energy), lipids (for building cell membranes), and amino acid-derived polypeptides and proteins (like enzymes). Among the molecules produced for export are hormones, which can be amines, polypeptides, proteins, or steroids. Some protein hormones also have carbohydrate components and are known as glycoproteins.
Hormones are generally classified into two groups based on their chemical properties: hydrophilic (water-loving, lipid-hating) and hydrophobic (water-hating, lipid-loving). Polypeptide and protein hormones usually fall into the hydrophilic category, while steroid hormones are typically hydrophobic. This distinction is important as it affects their synthesis, storage, release, transport in blood or other fluids, their receptor binding, and mechanisms of action.
However, there’s a third group of hormones that doesn’t neatly fit into these categories. This includes small, short-lived gaseous molecules like nitric oxide and carbon monoxide, and amines derived from amino acids such as iodothyronines and catecholamines. These hormones possess characteristics of both main groups, making them unique in their function and behavior in the body.
Polypeptide and Protein Hormones
These hormones are composed of amino acid chains. Polypeptides typically have 2–100 amino acids, while proteins consist of longer chains of over 100 amino acids. Some protein hormones are glycosylated, meaning they have carbohydrate residues attached, forming glycoproteins. They may also consist of multiple chains linked together. These hormones are usually first made as larger precursor molecules, known as prohormones, which are then cleaved to produce the active hormone(s).
The endocrine cells producing these hormones have specialized internal structures for processing and packaging them, including the endoplasmic reticulum, the Golgi complex, and secretory granules. The synthesis of these hormones starts with the activation of a specific gene within the cell’s nucleus. The human genome has about 30,000 genes, each coding for a specific protein. Gene activation and subsequent protein synthesis is referred to as gene expression.
A gene, a segment of DNA, carries the code for an amino acid chain. DNA strands, composed of nucleotides, form a double helix structure. During transcription, a specific enzyme called RNA polymerase initiates the creation of a messenger RNA (mRNA) molecule from the DNA template. This process involves the separation of the two DNA strands, with one strand serving as the template.
The transcription process starts at a special nucleotide sequence called the promoter and ends at another sequence called the terminator. DNA bases pair in a specific way, with adenine, cytosine, guanine, and thymine in DNA pairing with complementary bases in the RNA strand. Each three-nucleotide sequence in the RNA, called a codon, codes for a specific amino acid.
Not all sections of a gene are used for protein synthesis. Genes contain exons, which are transcribed into proteins, and introns, which are not. As the mRNA is synthesized, introns are removed by enzymes called small nuclear ribonucleoproteins (snRNPs or ‘snurps’). This process ensures that only the necessary parts of the gene are used to create the protein hormone.
The outcome of gene transcription is a functional strand of messenger RNA (mRNA), which exits the nucleus through a pore in the nuclear envelope. This active and selective transport of mRNA is an important step in protein synthesis. The initial mRNA synthesized from a gene can undergo alternative splicing, a process where it is split into multiple mRNA components, each potentially coding for a different protein.
The transition from an mRNA molecule to a new protein involves translating the coded information in the nucleotide sequence into a corresponding sequence of amino acids linked by peptide bonds. This translation happens in the ribosomes, which are either free in the cytoplasm or attached to the rough endoplasmic reticulum (RER), especially for proteins destined for export, like hormones.
Each ribosome comprises two subunits. The smaller subunit binds the mRNA, while the larger one has two sites for transfer RNA (tRNA) molecules. These tRNA molecules carry specific amino acids to the ribosome. The translation process starts when an mRNA molecule binds to a ribosome, and an initiator tRNA, complementary to the start codon on the mRNA, begins the protein synthesis. This initiator tRNA carries methionine, the starting amino acid for protein chains.
As translation progresses, each new tRNA brings its specific amino acid to the growing chain, with the amino acids being linked by peptide bonds. This process continues, following the mRNA code, until a stop codon is reached. Once the protein synthesis is complete, the newly formed protein detaches from the tRNA, and the ribosome disassembles.
The synthesis of a polypeptide hormone typically starts with the formation of a larger precursor protein known as a pre-prohormone. This pre-prohormone is synthesized on a ribosome and then enters the RER. Its entry into the RER is facilitated by a segment called the signal peptide, which is recognized and guides the molecule into the RER. This initial stage is crucial for the eventual formation and export of the hormone. For more detailed information on this process, specific textbooks on molecular biology provide comprehensive insights.
During the synthesis of peptide hormones, the initial step involves the creation of a pre-prohormone. Once this pre-prohormone enters the sacs or tubules of the rough endoplasmic reticulum (RER), a segment called the signal peptide is cleaved off, resulting in the formation of a prohormone precursor. This precursor then moves from the RER to the adjacent Golgi complex for further modification. Here, the prohormone can undergo several changes: it might be enzymatically cleaved to produce multiple proteins or polypeptides, glycosylated, or otherwise altered.
The final stage of peptide hormone synthesis involves packaging the breakdown products of the prohormone into vesicles. These vesicles bud off from the Golgi membrane and contain various peptide products, including the biologically active hormone itself. While many of these peptide products might not have a known function initially, ongoing research often reveals their roles or biological activities in the body. These vesicles serve as a crucial storage source for the hormone in protein hormone-secreting endocrine cells.
Interestingly, since all eukaryotic cells within an organism contain the same genetic material, and specific genes are typically expressed only in certain tissues, it’s possible for non-endocrine tissues to abnormally produce polypeptide hormones if the relevant gene is activated. This can occur when a cell is abnormally stimulated by certain chemicals, like carcinogens, causing inappropriate gene activation. As a result, non-endocrine tissues, such as lung tissue, can become abnormal (ectopic) sources of protein hormones. This situation can lead to complications, not only from the tumor growth itself but also due to the inappropriate, unregulated, and often excessively high levels of the bioactive hormone in circulation.
Steroid Hormones
Steroid hormones form another major group of hormones, all synthesized from cholesterol. These hormones are lipid-soluble (lipophilic), allowing them to move out of the endocrine cell upon synthesis. While earlier theories suggested that steroid hormones diffused through the cell’s lipid membrane, recent evidence points towards the involvement of specific transporters in the cell membrane that facilitate this process.
Due to their lipophilic nature, steroid hormones don’t accumulate much within their cell of synthesis; they tend to leave the cell almost as soon as they are produced. Therefore, steroid hormones are typically synthesized on demand, meaning they are produced when the endocrine cell receives the appropriate stimulus. The synthesis involves the activation of specific enzymes within the cell, which catalyze various chemical conversions such as hydroxylation and aromatization, transforming precursors into the final bioactive hormone molecules.
Like cells that produce peptide and protein hormones, cells that synthesize steroid hormones are also subject to a variety of stimulatory and inhibitory signals from the internal environment. These signals are integrated to determine the final endocrine response. In the case of steroid hormone-producing cells, the enzymes that convert the early molecular stages into the final bioactive hormone are the primary targets of these signaling pathways.
Amino Acid-Derived Hormones
Certain hormones, specifically those produced by the thyroid gland (like iodothyronines) and the adrenal medulla (such as catecholamines), are derived from amino acids and have unique synthesis pathways. The synthesis of these hormones begins with an amino acid, which undergoes various enzymatic modifications to become the final bioactive hormone.
The characteristics of these amino acid-derived hormones vary depending on the hormone type. They are typically stored within the endocrine cells or in follicular structures. When transported in the bloodstream, they may either circulate freely or be bound to proteins. These hormones exert their effects by binding to specific receptors located on the plasma membranes of their target cells, initiating a cascade of biological responses.
Eicosanoids: Prostaglandins, Thromboxanes, Prostacyclins, and Leukotrienes
Eicosanoids are a group of non-steroidal molecules that can be categorized as hormones due to their ability to traverse plasma membranes using specific transporters and are synthesized in response to specific stimuli. These include prostaglandins, derived from 20-carbon essential fatty acids found in most body cells. Prostaglandins are categorized into three series based on their precursors: Series 1 from gamma-linolenic acid, Series 2 from arachidonic acid, and Series 3 from eicosapentaenoic acid. The most well-known are those derived from arachidonic acid, which also include thromboxanes, prostacyclins, and leukotrienes. Collectively, these molecules are known as prostanoids.
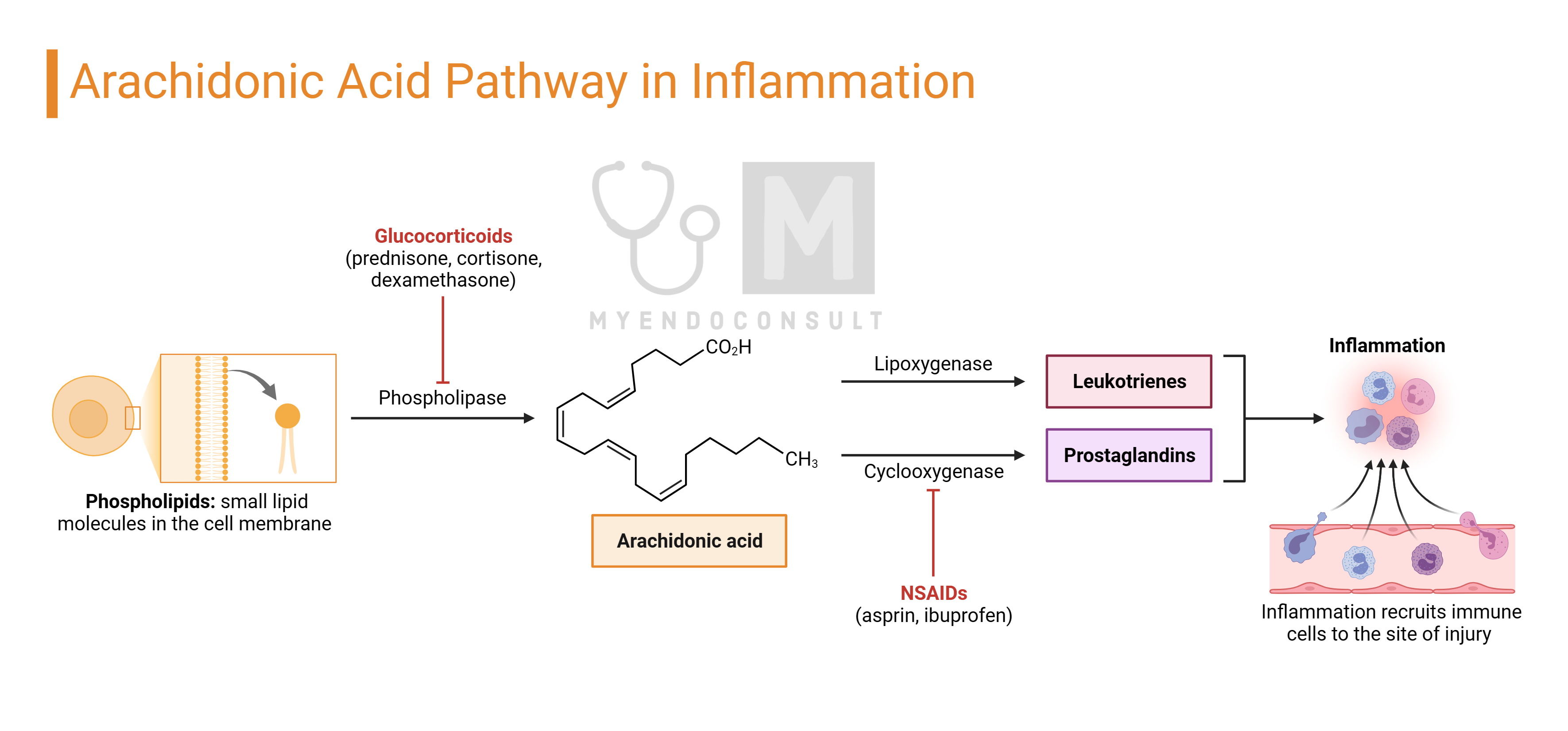
Prostanoids, which are ubiquitous lipids, exert physiological and sometimes pathological effects either within the cells where they are produced (autocrine action) or on nearby cells (paracrine action). They have very short half-lives and are not transported to distant cells, distinguishing them from classical hormones.
The synthesis of these molecules begins with the release of precursor substrates like arachidonic acid from cell membrane phospholipids, a process facilitated by specific enzymes such as phospholipase A2. Arachidonic acid is then converted by cyclooxygenases (COX1 and COX2) into intermediate prostaglandins like PGG2 and the unstable PGH2. These intermediates are quickly transformed into other prostaglandins (e.g., PGE2, PGF2α) by specific prostaglandin synthases. PGH2 also serves as a precursor for prostacyclin (PGI2) and thromboxanes, produced through the action of additional enzymes, prostacyclin and thromboxane synthases. These processes result in a variety of prostanoids, each with unique roles and actions within the body
Gaseous Molecules as Local Hormones
Nitric oxide (NO) and potentially carbon monoxide (CO) are gaseous molecules known to act as locally acting hormones. Due to their very short half-lives, measured in seconds, they affect only cells in close proximity to where they are produced.
NO was first identified as a significant biological molecule in the late 1980s, when it was discovered to be the endothelium-derived relaxing factor (EDRF) previously researched. This molecule, although essentially toxic in nature, was found to have numerous physiological roles, particularly in maintaining homeostasis and regulating the vasculature.
In the body, there are three types of nitric oxide synthases (NOS) responsible for producing NO: inducible NOS (iNOS) and two calcium-dependent constitutive enzymes found in vascular endothelial cells (eNOS) and nervous tissue (nNOS). In the vascular system, NO is synthesized from the amino acid L-arginine by the action of eNOS or iNOS. Once produced, NO diffuses from the endothelial cell to adjacent vascular smooth muscle cells, where it stimulates the conversion of guanosine triphosphate (GTP) into cyclic guanosine monophosphate (cGMP).
The activation of eNOS can be triggered by shear stress from blood flow against endothelial cells or via a receptor-activated pathway, both leading to the release of calcium ions from intracellular stores. This increase in intracellular calcium ion concentration activates eNOS. iNOS, in contrast, is activated by longer-term inflammatory responses. The produced NO then activates guanylate cyclase in the adjacent smooth muscle, leading to cGMP synthesis. cGMP induces muscle relaxation by activating potassium channels, causing membrane hyperpolarization, and reducing calcium entry into the cell. This results in decreased calcium-calmodulin formation, reducing myosin-actin binding and thereby causing muscle relaxation. cGMP also stimulates myosin light chain phosphatase, which further contributes to smooth muscle relaxation.
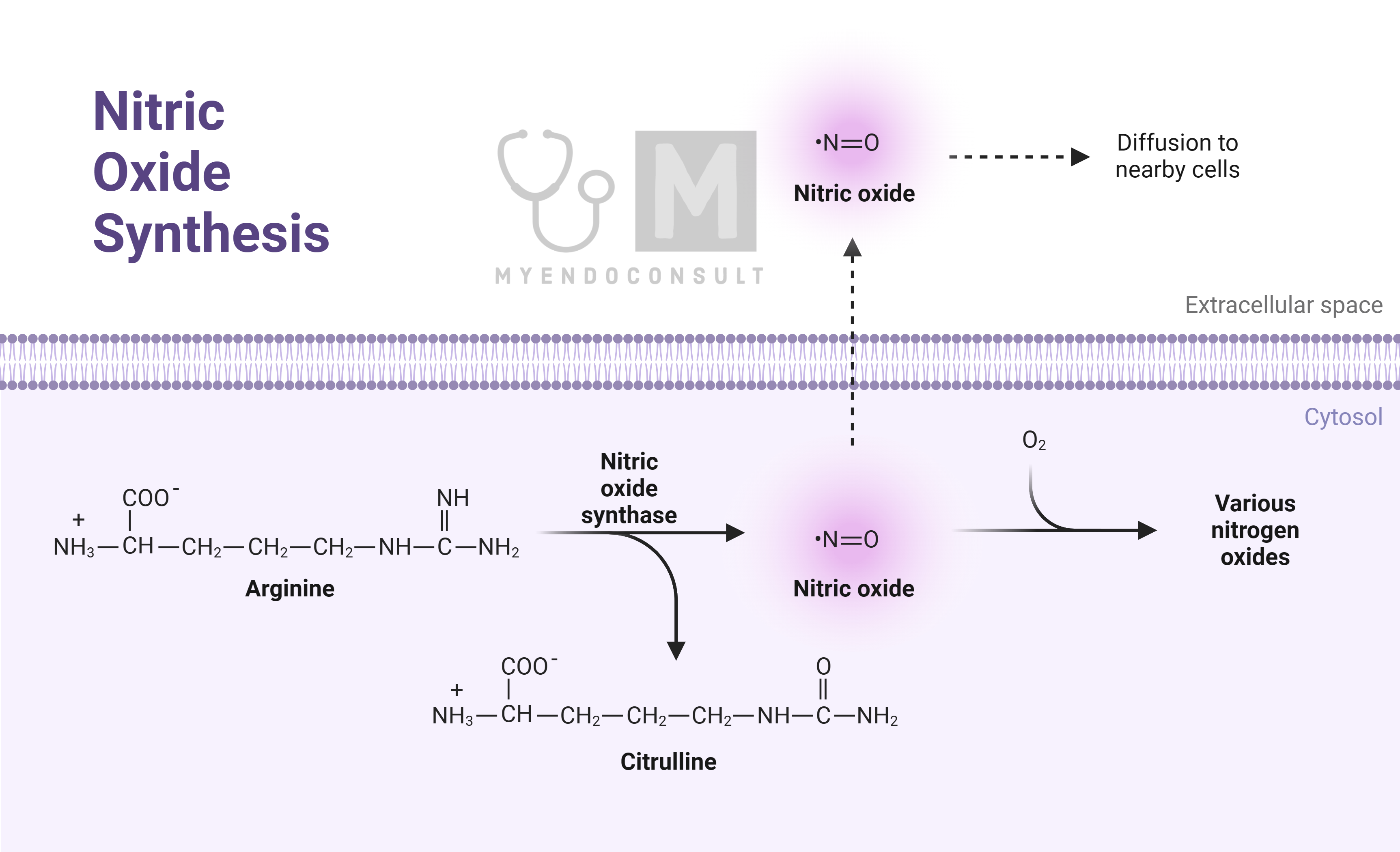
NO has a short lifespan due to rapid inactivation by superoxides, which are reactive oxygen species (ROS) produced within the cell. This brief effective period restricts its impact to the immediate vicinity of its production site.
Kindly Let Us Know If This Was helpful? Thank You!